Photochemistry
The title of this article is ambiguous. For photochemistry used to produce photographic images, see development (photography).
The term photochemistry refers to chemical reactions that are initiated by the action of light. The basic prerequisite for this is an absorption of the light by the molecule that is to react. This means that the wavelength of the light used must match the absorption behaviour of the molecule. In addition to direct excitation, there are also photoreactions in which a photosensitizer is first excited and this then transfers energy to the molecules to be made to react.
The absorption of a light quantum leads to energetically (electronically) excited states, which can enter into chemical reactions thanks to the excitation energy. Chemical transformations thereby compete with photophysical deactivation processes such as photoemission from the excited singlet state (fluorescence) or from the triplet state (phosphorescence) as well as non-radiative deactivation. The relative extent to which each process is undergone is expressed by the quantum yields. The sum of the quantum yields is 1 - except for chain reactions.
Since the absorption of a light quantum leads to an electronic excitation, reactions can be observed in the excited state that are not allowed in the electronic ground state of the molecule (cf. Woodward-Hoffmann rules, pericyclic reactions). Photochemical reactions are often a good method to build complex and highly strained molecules.
In addition to the action of light, photochemistry can also be carried out with higher-energy quanta. Among other things, X-ray photochemistry or high-energy photochemistry deals with such photoinduced processes. Here, synchrotron radiation is used in chemistry.
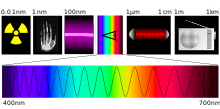

Light as part of the electromagnetic spectrum
_V.1.svg.png)

Homolytic cleavage of molecule A-B under the influence of light. Two radicals are formed (right).
Examples of photochemical reaction types
- Cleavages (bond homolyses), as observed e.g. with photoinitiators - possibly followed by further fragmentation of the resulting radicals, cf. the cleavage of carbon monoxide from carbonyl compounds.
- Photoisomerizations, such as the formation of fulvene and benzvalene from benzene via the first excited singlet state or the formation of dewarbenzene from the second excited singlet state of benzene.
- Electrocyclic reactions, such as the conversion of ergosterol to previtamin D, the cyclization of butadienes to cyclobutenes, the cyclization of cis-stilbene to dihydrophenanthrene, or of diphenylamine to dihydrocarbazole.
- Rearrangements, such as the isomerization of cycloheptatriene to toluene.
- Light-induced chain reactions:
- Reaction of chlorine and hydrogen (chlorine oxyhydrogen gas) to hydrogen chloride, the reaction mechanism of which was elucidated in particular by Walther Nernst and Max Bodenstein.
- Photochlorination of alkanes, e.g. methane.
- Regioselective side-chain halogenation of alkylated aromatics (simplest example: chlorination of toluene at the methyl group) according to the "SSS" rule (sun, boiling heat, side chain).
- Sulfochlorination of alkanes with sulfur dioxide and chlorine.
- Photo-Fries shift of phenyl esters to form ketones with a hydroxy function in the ortho and/or para position on the phenyl ring.
- [2+2]-cycloadditions of alkenes in the course of photodimerization or photocyclization leading to cyclobutanes (four-ring), cf. the formation of quadricyclane by exposure of norbornadiene or the photodimerization of cyclopentene.
- [2+2]-cycloaddition of alkenes and ketones in the Paternò-Büchi reaction.
- α-Cleavage of thiol esters to aldehydes and disulfides.
- Formation of thioxanthones by rearrangement reactions.
- isomerizations, such as cis-trans isomerizations (standard examples: cis/trans-stilbene, cis/trans-azobenzene, maleic/fumaric acid). For example, irradiation of maleic acid and fumaric acid, respectively, produces the same mixture of 75% maleic acid and 25% fumaric acid in both cases. The position of the photoequilibrium can be controlled by the excitation wavelength.
- Photosensitized reactions, i.e. photoisomerizations, photocycloadditions, with addition of a photosensitizer, some of which are enantioselective.
- Photoreactions in biology:
- A well-known example of a chemical reaction with photochemical reaction steps is photosynthesis.
- Another example is vision with the human eye. In the rods of the retina of the eye, a photoisomerization of the rhodopsin responsible for light-dark vision takes place, more precisely, a light-controlled cis-trans isomerization of the 11-cis-retinal, which is a component of the chromophore rhodopsin.
The first photochemical experiments can be traced back to Giacomo Luigi Ciamician. The first summary books on preparative organic photochemistry were written by Alexander Schönberg.
- A photophysical process in biology:
- Fluorescence quenching with the help of quenchers such as carotenoids (carotene in carrots, lycopene in tomatoes), which occur in plants as antioxidants and prevent the formation of the toxic singlet oxygen.
Performance of photochemical experiments
The performance of photochemical experiments requires a number of prerequisites that result from the starting condition - absorption of light by the reactants. It must be known at which wavelength the photochemical excitation is to take place. Corresponding information on the reactants can be obtained from tables or by measuring the UV-VIS spectra. The next step is to ensure that a suitable light source is available. It must be ensured that the light source provides sufficient power in the relevant wavelength range. It may also be necessary to exclude wavelengths that lead to photochemical side reactions. The solvents used must be transparent in the relevant wavelength range (unless they act as sensitizers for the photoreaction). Furthermore, the solvents must not act as "quenchers" (take over singlet or triplet excitation energy of the reactants and thus deactivate the reactive species) and must be inert towards the species occurring. Oxygen usually leads to side reactions, which is why the reactions are usually carried out under protective gas (nitrogen, argon) (exception: reactions of singlet oxygen, in which oxygen is specifically passed through the reaction mixture). Since the penetration depth of the light is usually only a few millimeters (cf. Lambert-Beer's law), good mixing must be ensured. In laboratory tests, this can often be achieved by passing inert gas through the chamber, which is necessary anyway. Protection against UV radiation (eye damage, "sunburn") or the dissipation of the high amounts of heat generated, e.g. by high-pressure lamps, is relevant from a safety point of view.