Nuclear power
This article presents the generation of electric power from nuclear fission. For the generation of electric current from nuclear fusion see fusion energy
Atomic power is a redirect to this article. For the British music group see Atomkraft (band)
Nuclear energy, atomic energy, atomic power, nuclear power or nuclear energy is the technology for the large-scale generation of secondary energy by means of nuclear fission. This technology has been used on a large scale to produce electricity since the 1950s.
As of January 2020, 447 reactor units with a total capacity of 397 GW were in operation in 31 countries. A further 53 reactor units with a total capacity of 54.7 GW are under construction. In addition, more than 100 nuclear power plants are planned for the coming decade. Since 181 nuclear power plant units have already been shut down in parallel with the new builds, the number of reactors has remained largely constant since the 1990s. As a result of rising electricity generation worldwide, the share of nuclear power fell from 17.5% to 10.15% from 1996 to 2018. In 2018, the electrical energy generated by nuclear power plants was a good four percent lower than in 2006, when it reached a historic high. In the EU, nuclear power plants cover about 25% of the electricity consumed (787 TWh of generation with 117 GW of installed capacity).
In addition to stationary nuclear reactors, there are about 180 reactors on about 140 watercraft, including nuclear submarines, aircraft carriers, some nuclear icebreakers, 4 cargo ships, and a sea-based nuclear power plant. Satellites with nuclear reactors have also been operated and reactor concepts for aircraft have been researched.
There are different views on the advantages and disadvantages of nuclear fission energy. The use of nuclear fission energy in general as well as its safety in particular are controversially discussed in science and in the public.
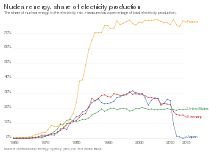

Share of electricity generation from nuclear energy per country .
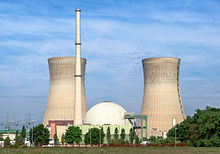

The Grafenrheinfeld nuclear power plant, between the cooling towers the concrete dome with the nuclear reactor
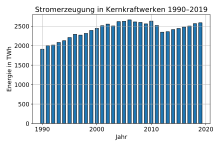

Worldwide electricity generation in nuclear power plants 1990-2019 in TWh
History
Conceptual History
In 1899, the physicist Hans Geitel was one of the first to coin the term atomic energy for the phenomena occurring in connection with radioactive decay processes. Later, the synonyms atomic energy, atomic power, nuclear power and nuclear energy were added.
The use of these terms has undergone a politically and ideologically motivated shift. In the 1950s, Franz Josef Strauß was the Federal Minister for Atomic Affairs. A conference held in Geneva in 1955 with high-ranking scientists was entitled International Conference on the Peaceful Uses of Atomic Energy and became known in the German media as the Atomic Conference. As a result of this conference, the International Atomic Energy Agency (IAEA) was founded in 1957. The lobbying association of German companies interested in the technology was founded in 1959 as the German Atomic Forum. In the following decades, proponents of the technology distanced themselves from the prefix atom and used only nuclear in Germany. Parallel to this, a shift from atomic to nuclear occurred in the English-speaking world. The reason for this was the undesirable association with the increasingly negative concept of the atomic bomb; the technical-physical justification emphasised that the relevant processes take place in the nucleus and not in the entire atom, whose chemical properties are determined by the atomic shell. Critics, on the other hand, retained the prefix atom both in the self-designation anti-nuclear and in slogans such as "Nuclear power? No thanks". They continued to speak of nuclear energy and nuclear power plants with the abbreviation AKW.
The synonym atomic nuclear energy was used in the first period of technical use (name change of the Ministry of Atomic Energy to the Federal Ministry of Atomic Nuclear Energy in 1961) and until today as a nuclear legal term, for example at the Länder Committee for Atomic Nuclear Energy.
History of Technology
→ Main article: Discovery of nuclear fission
Around 1890, the first experiments on radioactivity were carried out. The aim of Antoine Henri Becquerel, Marie and Pierre Curie and others was to study nuclear reactions.
In 1938, Otto Hahn and Fritz Straßmann discovered the induced nuclear fission of uranium, which was theoretically explained by Lise Meitner and Otto Frisch in 1939. Together with the demonstration, in particular by Frédéric and Irène Joliot-Curie, that a chain reaction is possible because each nuclear fission triggered by a neutron releases several more neutrons, the practical applications of nuclear fission became clear.
These findings were first used for military research during the Second World War. As part of the Manhattan Project, Enrico Fermi achieved the first controlled nuclear chain reaction in a nuclear reactor in Chicago (Chicago Pile One) on 2 December 1942. While the goal of the Manhattan Project led by Robert Oppenheimer was achieved with the first successfully detonated atomic bomb on 16 July 1945 (Trinity Test), the German research group under Werner Heisenberg and Carl Friedrich von Weizsäcker did not succeed in developing a functioning nuclear reactor until the end of the war (Uranium Project).
After the Second World War, military research continued. In parallel, the civilian use of nuclear energy was developed. At the end of 1951, the experimental reactor EBR-I in the US state of Idaho generated electrical power from nuclear energy for the first time and lit four light bulbs on 20 December. The first power plant for the large-scale generation of electrical energy was put into operation in 1954 with the Obninsk nuclear power plant near Moscow. This was followed in 1955 by the Calder Hall nuclear power station in north-west England on the site of the Sellafield nuclear complex.
In Germany, the first research reactor, the Munich Research Reactor in Garching, went into operation in 1957. In 1961, electricity from nuclear energy was fed into the West German supply network for the first time from the Kahl nuclear power plant with a capacity of 15 MW on the territory of the municipality of Karlstein am Main. In 1966, the Rheinsberg nuclear power plant went into operation in the GDR. The expansion of nuclear energy in West Germany was not market-driven or a reaction to an energy shortage. Instead, the key role was played by state authorities, while the energy supply companies, for example, were "for a long time the braking factor in the implementation of nuclear energy". It is even argued that in the early years the key driver for the German nuclear energy programme was to create the option of nuclear weaponry. While German nuclear policy, in continuation of the nuclear project during the Nazi dictatorship, initially focused on the heavy water reactor, in the 1960s the more favourable American concept of the light water reactor was adopted, a "victory of the economists over the technicians".
This imitation of the Americans resulted in specific problems for Germany: In view of the USA's status as a nuclear power, the civilian American reactors were chosen in such a way that they benefited from the military uranium and plutonium plants, which meant that a fluid boundary to military technology was a basic prerequisite for reactor development there. Therefore, the suitability of American reactor technology for Germany was questionable in this respect, at least in the case that Germany would have considered itself a non-nuclear power for all times. In addition, the safety philosophy on both sides of the Atlantic was different: In the USA, people were aware that light water reactors offered less inherent safety than other reactor types discussed at the time. Therefore, there was a broad consensus that one had to expect the worst and that nuclear power plants should accordingly be built mainly in sparsely populated regions that could be easily evacuated. In the much more densely populated Federal Republic of Germany, on the other hand, this was not possible, as otherwise it would hardly have been possible to designate reactor sites. Instead, in order to be able to avoid a corresponding safety distance, it was considered to build nuclear power plants underground, but this was vehemently rejected by the nuclear industry. Other plans, on the other hand, envisaged nuclear power plants located close to the cities of Ludwigshafen am Rhein and Frankfurt am Main in order to supply the chemical industry there with process heat; this was largely motivated by the desire to prove the special reliability of German nuclear power plants.
In the 1960s, numerous other nuclear power plants with significantly higher output were built. For example, the Gundremmingen nuclear power plant, which went into operation in 1966, had a capacity of 250 MW. In 1968, the ore freighter "Otto Hahn" was commissioned as a nuclear-powered research freighter; after the end of nuclear operation in 1979, it was converted back to diesel propulsion.
In the 1970s, especially after the first oil crisis in 1973, the construction of nuclear power plants was pushed. These nuclear reactors, such as Unit B of the Biblis nuclear power plant, have a capacity of about 1.3 GW (= 1300 MW). In the course of the anti-nuclear movement's protests against the construction of the Wyhl nuclear power plant in Germany in 1975, a larger opposition to the civilian use of nuclear energy emerged. In Austria, a referendum in 1978 decided not to put the Zwentendorf nuclear power plant, which had already been completed, into operation. Criticism of nuclear power intensified and intensified in particular as a result of the serious reactor accident at the Three Mile Island nuclear power plant near Harrisburg (USA) on 28 March 1979, in which a partial core meltdown occurred for the first time.
In 1983, the thorium high-temperature reactor (THTR-300 nuclear power plant) was put into operation in Schmehausen. It goes back to the developments by Rudolf Schulten. This prototype pebble bed reactor was decommissioned six years later after several technical malfunctions, long shutdown phases and only 14 months of full load operation. The decommissioning had become necessary because the plant was on the verge of insolvency in 1989 and no agreement could be reached on taking over the high operating losses that were still to be expected. The THTR was transferred to safe enclosure.
On 26 April 1986, the Chernobyl disaster occurred, in which large quantities of radioactivity also fell in Western Europe after a core meltdown. As a result, criticism of the use of nuclear energy increased significantly, particularly in Europe. In 2000, under pressure from the German government, it was decided to phase out the commercial use of nuclear energy by around 2020. Within this framework, two nuclear power plants were taken off the grid by 2005. In 2010, the black-yellow coalition Merkel II cabinet decided to extend the operating lives of German nuclear power plants by 8 and 14 years, respectively. This decision was highly controversial politically and socially (even more so since the Fukushimanuclear disaster in Japan since March 2011). The serious accident in Fukushima showed that the light water reactor with Mark-I containment from General Electric, which is widely used worldwide, does not conclusively meet the requirements for safety, whereby the reasons for the accident also lay in wrong decisions on the part of the operator (economic efficiency before safety) and negligence on the part of the authorities. As a reaction to this, the German government initially announced a three-month nuclear moratorium in March 2011; finally, the nuclear consensus decided to phase out nuclear power by 2022, and the eight oldest nuclear power plants were shut down immediately.
International perspective
→ Main article: Nuclear energy by country
According to IAEA/PRIS, the number of nuclear power plants and installed capacity have increased since 1995. The number of reactor units increased from 434 to 445, while available capacity rose from 341 GW to 387.4 GW. In 2011, 65 reactors were under construction worldwide and 114 were in planning, according to the IAEA. However, after the Fukushima nuclear disaster, many new construction plans were revised. China, for example, temporarily halted all new construction plans.
In the US, President Barack Obama announced the construction of a "new generation of safe, clean nuclear power plants" and offered government loan guarantees of €38.6 billion. As of February 2020, two nuclear reactors are under construction in the US.
The former French government under François Fillon reaffirmed in 2011 that nuclear energy would remain the cornerstone of the 40-year policy of energy independence. President Hollande, newly elected in May 2012, agreed with the (French) Greens during the election campaign to shut down 24 of France's 58 reactors.
As of February 2020, seven nuclear power plants are being built in India. It is planned to cover 25 % of the electricity demand by nuclear energy by 2050.
As of February 2020, 10 nuclear power plants are under construction in China, and about 148 more reactor units are in the planning stage. In March 2011, the government temporarily suspended the approval of new nuclear power plants. By 2020, the installed capacity was expected to increase eightfold to a total of 80 GW, but by February 2020, only 45.53 GW had been installed. In July 2011, it was reported that China would again focus on a rapid expansion of nuclear power. The Fukushima nuclear disaster had not changed this much.
As of February 2020, Russia operates 38 reactors and is building 4, with 31 in planning.
South Korea formerly pushed nuclear power, currently 5 reactors are still under construction. In June 2017, South Korean President Moon Jae-in announced his intention to completely phase out nuclear power by 2057. In the future, the existing nuclear power plants are to be taken off the grid after 40 years; the oldest reactor unit, Kori 1, was accordingly shut down on 18 June 2017.
Following a referendum in June 2011, in which 95 % of the citizens decided against re-entry, Italy shelved the re-entry planned by the Berlusconi government.
The Czech Republic has scrapped plans to build new nuclear power plants. The tender for the two new nuclear power plant units at the Temelín site has been withdrawn by the semi-state-owned energy company ČEZ.
Germany already decided under the Schröder government to phase out nuclear power by around 2020/21. The Merkel government initially extended the lifetimes, but initiated an accelerated nuclear phase-out by 2021 after the reactor accident at Fukushima.
In Japan, all reactors were gradually taken off the grid for maintenance reasons by 5 May 2011. The local parliaments are responsible for restarting the reactors, and for a long time they have rejected all applications. In mid-September 2012, a year and a half after the Fukushima nuclear disaster, the Japanese government decided to phase out nuclear power by 2040 at the latest. However, the government of Shinzo Abe has reversed this phase-out. On 10 August 2015, the Sendai nuclear power plant became the first nuclear power plant to restart after the Fukushima nuclear disaster. Applications for re-licensing were underway for a further 25 reactors. After the shutdown of the nuclear power plants, electricity prices increased by 20 to 30%; Japan had to import fossil fuels for an estimated additional €26 billion in the year. By 2019, 8 reactors had come back online, 21 were permanently shut down, and 25 are officially operating, although most will never come back online. For example, Fukushima II had been out of service since March 2011, but was not officially shut down for good until September 30, 2019.
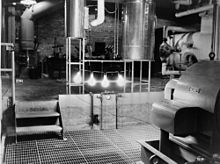

The four incandescent lamps in the EBR-I experimental reactor on 20 December 1951
![Installations and deinstallations of nuclear power plants for electricity generation. (Output of all newly installed nuclear power plants [solid frame] or all destroyed or permanently decommissioned nuclear power plants [dotted frame] - broken down by year and country. The legend indicates the ISO-3166-1 codes of the countries. Source:)](https://alegsaonline.com/image/310px-Kernenergie_nach_Jahren.svg.png)

Installations and deinstallations of nuclear power plants for electricity generation. (Output of all newly installed nuclear power plants [solid frame] or all destroyed or permanently decommissioned nuclear power plants [dotted frame] - broken down by year and country. The legend indicates the ISO-3166-1 codes of the countries. Source:)
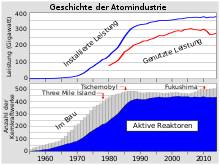

The number of active reactors has stagnated since 1990
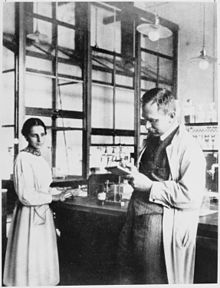

Lise Meitner and Otto Hahn in the laboratory, 1913
Technologies
The use of nuclear energy requires not only nuclear power plants, but also mines to extract uranium ore, uranium enrichment plants to convert the ore into nuclear fuel, interim storage facilities to safely store spent fuel rods, nuclear chemical plants to prepare the resulting radioactive waste for long-term storage, and finally repositories to store the radioactive waste until it is rendered harmless by radioactive decay. If the post-fission parts of the uranium and newly created fissile elements in spent fuel rods are to be used for the further generation of electrical energy in nuclear power plants, then additional reprocessing facilities are used.
Nuclear Fission
→ Main article: Nuclear fission
In induced nuclear fission, an atomic nucleus of a uranium or plutonium isotope decays into (usually) two lighter nuclei (the fission fragments) after absorbing a neutron. The energy released comes from the difference in binding energy of the fission fragments compared to the original nucleus and is released in the form of kinetic energy of the fission fragments and as gamma radiation. Including the energy that is still released during the subsequent radioactive decay of the fission fragments, this results in about 200 MeV per fission, i.e. just under 1 MeV per nucleon. In addition to the fission products, 2-3 prompt neutrons are also released during fission. These can cause further nuclear fissions and thus lead to a chain reaction. The delayed neutrons still emitted from the fission fragments after fission make it possible to technically control the chain reaction in a nuclear reactor (see criticality).
The energy yield of about 200 MeV per fission corresponds to a thermal energy of about 0.96 MWd (megawatt-days) per gram of uranium-235 or plutonium-239. The same thermal energy can be obtained by burning 2.8 t of hard coal, 10 t of lignite or 1.9 t of light fuel oil.
Nuclear power plant
→ Main article: Nuclear power plant
Nuclear power plants convert the energy from nuclear fission into thermal energy and this into electrical energy. Nuclear power plants carry out controlled chain reactions of nuclear fission in nuclear reactors. The heat released by this process is used to produce steam, which is directed to turbines that drive generators, producing electrical power. Furthermore, in the military sector, some aircraft carriers, nuclear submarines and a few nuclear cruisers are equipped with nuclear propulsion; in the civilian sector, this propulsion has only become established for nuclear icebreakers (see also: List of civilian ships with nuclear propulsion).
Security
→ Main article: Reactor safety
The safety of nuclear fission power plants is playing an increasingly important role, especially as a result of the Chernobyl and Fukushima reactor accidents, in which the chain reaction and residual heat production, respectively, got out of control. The increasingly stringent safety regulations led to many additional safety measures, but also to increased operating costs.
Fuel chain
→ Main article: Fuel cycle and uranium economy
The generic term fuel cycle is commonly used for the totality of steps involved in supplying nuclear reactors with fuel elements, including the necessary measures for the disposal of radioactive waste. This term originally became known in the discussion about the construction of the Wackersdorf reprocessing plant. The term "cycle" does not mean complete recycling of the material; in reprocessing plants, the used fuel is to be broken down into its components after removal from the reactor, thus producing starting material for new fuel elements.
Range of fuels
→ Main article: Uranium mining
World uranium deposits by price category for mining, excluding predicted and speculative deposits. Status 2014 | ||||
Price category | secured | suspects | totally | Range |
$/kgU | kt | kt | kt | Years |
<40 | 507 | 176 | 683 | 11 |
40-80 | 1.212 | 745 | 1.957 | 31 |
80-130 | 3.699 | 2.204 | 5.902 | 95 |
130-260 | 4.587 | 3.048 | 7.635 | 123 |
total: | 16.178 | 261 |
Similar to fossil fuels, the Earth's supplies of nuclear fuel are limited. The table gives an overview of the known mineable uranium reserves and uses the current consumption of just under 62,000 tU/year. Currently (7/2016), the price of uranium is about $60/kg. Since uranium only accounts for a fraction of the value chain of nuclear energy (according to the information letter of the 'Wissenschaftliche Dienste des Deutschen Bundestages' about 5 %), even a multiplication of the uranium price would only have a minor impact on the total costs and thus on the electricity price.
The use of thorium (Th232) as nuclear fuel is proposed by various parties. However, thorium is not fissile; it must first be converted into fissile U233 in an incubation process, similar to the use of U238. Thorium, at 9.6 ppm, is about three times more abundant in the earth's crust than uranium, at 2.7 ppm, of which only U235, at 0.7% by weight, has been used to date. With the aid of this breeder technology, which has not yet been used on a large scale, the range given in the table could thus be extended by a factor of about 100 (U238) or 300 (thorium).
Since nuclear fuel is no longer mined in Germany, German nuclear power plants are dependent on imports.
Extraction and enrichment
→ Main article: Uranium enrichment
After mining, the ore is ground and the uranium is chemically extracted, usually as triuranoctoxide (U3O8). The U3O8 is then converted into gaseous uranium hexafluoride (UF6). While uranium with the natural isotope distribution of 99.3 % 238U to 0.7 % 235U can be used in heavy water reactors and in breeder reactors, the widely used light water reactors require enriched uranium with a proportion of up to about 6 % 235U. Enrichment of 235U is usually accomplished by gaseous diffusion or ultracentrifugation of uranium hexafluoride. The uranium enriched in U-235 is then processed into fuel rods as uranium dioxide, possibly together with plutonium dioxide as mixed oxide. Several fuel rods are then combined to form fuel assemblies.
About half of uranium mining currently takes place in sparsely populated areas of Kazakhstan, Canada and Australia. Uranium and thorium are mostly extracted in mining operations for other more common metals, such as the Olympic Dam mine in Australia. The uranium content of currently exploited deposits varies considerably, from 0.03 to 18 percent. Uranium mining in the former GDR, namely at Wismut, was historically important for weapons production.
During the operation of nuclear reactors, fissile plutonium is produced by the irradiation of uranium with neutrons. It is recovered from the spent fuel during reprocessing and can be used for new fuel elements. Another currently (2016) significant source is uranium and plutonium from former nuclear weapons that have been decommissioned as a result of disarmament.
The World Nuclear Association believes it is possible to increase uranium reserves by extracting it from coal-fired power plant ash.
- Online calculator for uranium enrichment (WISE Uranium Project)
Interim storage
→ Main article: Interim storage facility
Nuclear fission produces many different radioactive isotopes. Therefore, spent fuel elements radiate strongly; their material must not be released into the environment. Immediately after use, the radiation is so strong that further processing is not possible. For this reason, the fuel elements are stored for several months or years in the interim storage facility of the nuclear power plant in a decay pool. After this time, short-lived isotopes have largely decayed. However, long-lived isotopes remain, which means that the fuel elements are still highly radioactive and also continuously produce heat. Some of these isotopes are fissile and can, in principle, be used as nuclear fuel after chemical separation. The rest must be stored until it is rendered harmless by radioactive decay.
Special transport casks, such as Castor casks, are used to transport and store the fuel elements outside the decay pool. Spent, non-reprocessed fuel elements and radioactive waste from reprocessing plants are stored in storage casks in interim storage facilities until the heat generation has decayed to such an extent that final disposal is possible. This takes several decades.
Reprocessing
→ Main article: Reprocessing
In reprocessing plants - such as the La Hague reprocessing plant in France - the 95 % uranium and 1 % plutonium contained in spent fuel can be separated from the 4 % fission products and higher actinides. Of the separated uranium, about 10% can be reused after re-enrichment (e.g. in Seversk, Russia). 90 % is still radioactive waste. However, the proportion of high-level waste is decreasing considerably (e.g. 5%). According to research by Greenpeace, La Hague discharges around 0.5 billion litres of radioactively contaminated wastewater into the English Channel each year, as well as releasing radioactively contaminated exhaust air over Europe. The proportion of radioactive krypton (85Kr) in the exhaust air (approx. 90,000 Bq per cubic metre of air) is particularly significant.
In Germany, a reprocessing plant was under construction at Wackersdorf, but was not completed for financial reasons and because of strong opposition from the public.
One way of converting long-lived radioactive waste is to transmute it into isotopes that are either stable or whose radioactivity has decayed to harmless levels in a few hundred years. These facilities are currently under research and development and are supported at European level by the Eurotrans project. Technically, these facilities consist of a proton accelerator system and a target in which fast neutrons are produced. Some of the problematic isotopes can be transmuted into less problematic isotopes by bombarding them with fast neutrons. However, transmutation requires multiple, complex reprocessing steps. The first experimental facility is to be built in 2020 as part of the Myrrha project in Belgium.
Final disposal
→ Main article: Repository
The radioactive waste from a nuclear power plant continues to radiate strongly even after decades. Highly radioactive waste (High Active Waste) has only decayed sufficiently after a few thousand to a few hundred thousand years (depending on what is considered to be harmless). In addition, some elements contained in it are also chemically very toxic. Therefore, radioactive waste must be stored in a repository in such a way that it is permanently kept away from the biosphere. Highly radioactive waste must first have decayed to such an extent that the decay heat can no longer lead to weakening of the storage container (e.g. through corrosion). This is done in interim storage facilities, which can be designed as dry or wet storage facilities. In Germany, dry storage is common; the steel storage containers - for example castors - are stored upright and have cooling fins.
In 2015, the Finnish government granted the construction permit for the world's first repository for high-level radioactive waste, the Onkalo repository in Olkiluoto. Storage is scheduled to begin in 2020.
Decommissioning of a nuclear power plant
At the end of the service life of a nuclear power plant after about 40 years, the decommissioning and dismantling of the nuclear power plant takes place. According to the IEA, around 200 nuclear power plants will be decommissioned by 2024 due to their age. The IEA estimates that the cost of decommissioning these nuclear power plants will be over $100 billion. The expense is so high because almost all components are radioactive, must be separated and stored according to their half-life.
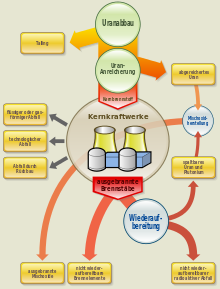

Fuel cycle with supply, disposal and reprocessing
Questions and Answers
Q: What is nuclear power?
A: Nuclear power is the controlled use of nuclear energy to generate electricity.
Q: How does a nuclear reactor work?
A: A nuclear reactor uses nuclear reactions to release energy which then boils water and powers a steam engine, producing electricity.
Q: What percentage of the world's electricity came from nuclear power in 2007?
A: In 2007, 14% of the world's electricity came from nuclear power.
Q: What are some potential risks associated with using nuclear power?
A: Nuclear power plants produce radioactive waste that could be harmful if it is not stored properly.
Q: What type of alternative energy source has been studied since the middle 20th century?
A: People have been studying fusion power since the middle 20th century as an alternative energy source.
Q: How does fusion power differ from traditional nuclear power?
A: Fusion power produces much more energy than traditional nuclear power and doesn't produce any radioactive waste.
Q: Are fusion reactors available yet?
A: Fusion reactors do not exist yet and are still being developed.