Atom
This article is about the scientific atom - for other meanings, see Atom (disambiguation).
Atoms (from the ancient Greek ἄτομος átomos "indivisible") are the building blocks of which all solid, liquid or gaseous substances are composed. All the material properties of these substances, as well as their behavior in chemical reactions, are determined by the properties and spatial arrangement of their atoms. Each atom belongs to a particular chemical element and forms its smallest unit. At present, 118 elements are known, of which about 90 occur naturally on Earth. Atoms of different elements differ in size and mass, and especially in their ability to react chemically with other atoms and combine to form molecules or solids. The diameters of atoms range from 6 - 10-11 m (helium) to 5 - 10-10 m (caesium), their masses range from 1.7 - 10-27 kg (hydrogen) to just under 5 -10-25 kg (the heaviest nuclei currently produced synthetically).
Atoms are not indivisible, as assumed at the time of naming, but show a well-determined structure of even smaller particles. They consist of an atomic nucleus and an atomic shell. The atomic nucleus has a diameter of about one tenth to one hundred thousandth of the total atomic diameter, but contains over 99.9 percent of the atomic mass. It is composed of positively charged protons and a number of electrically neutral neutrons of approximately equal weight. These nucleons are bound together by the strong interaction. The shell consists of negatively charged electrons. It contributes less than 0.06 percent of the mass, but determines the size of the atom. The positive nucleus and the negative shell are bound together by electrostatic attraction. In the basic electrically neutral form of the atom, the number of electrons in the shell is equal to the number of protons in the nucleus. This number determines the exact structure of the shell and thus the chemical behavior of the atom and is therefore called the chemical atomic number. All atoms of the same element have the same chemical atomic number. If additional electrons are present or missing, the atom is negatively or positively charged and is called an ion.
The idea of the atomic structure of matter already existed in antiquity, but was controversial until modern times. The final proof could only be provided at the beginning of the 20th century and is considered one of the most important discoveries in physics and chemistry. Individual atoms cannot be seen even with the most powerful light microscopes. Direct observation of individual atoms has only been possible since the middle of the 20th century with field ion microscopes, and for some years now also with scanning tunneling microscopes and high-resolution electron microscopes. Atomic physics, which studies not only the structure of atoms but also the processes inside them and their interactions with other atoms, has made a decisive contribution to the development of modern physics and, in particular, quantum mechanics.
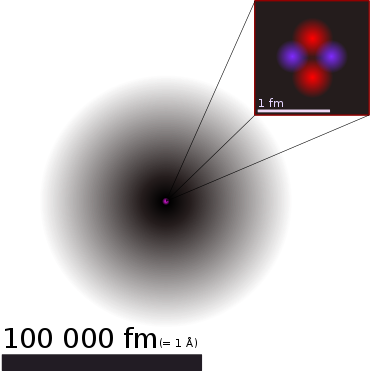

A helium atom: The atomic nucleus (pink) lies in the centre of a much larger cloud of two electrons (grey). In a scale representation, an atomic nucleus with a diameter of 1 millimetre would have an electron cloud of about 100 metres. Right side up, nucleus of each two protons and neutrons is drawn additional schematic and enlarged. In reality, the arrangement of the four particles is spherically symmetrical.
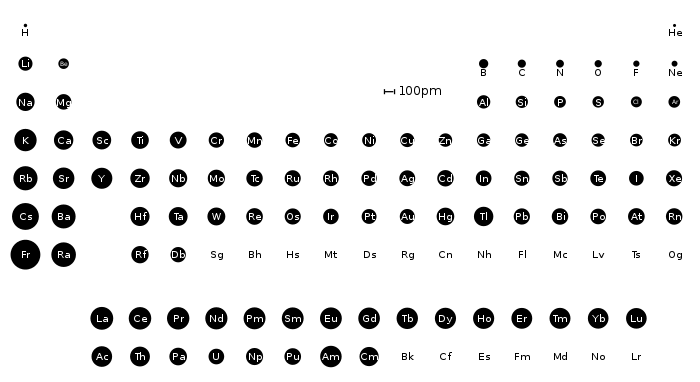

The atoms of the elements in the arrangement of the periodic table in scale representation of their covalent radius
Exploration History
The idea of the atomic structure of matter already existed in antiquity, but only in the form of philosophical considerations. Even at the beginning of the 20th century, its existence was disputed. Due to their extremely small size, individual atoms cannot be detected even with the most powerful light microscopes. The definitive proof of their existence is considered one of the most important discoveries in physics and chemistry. Albert Einstein made a decisive contribution in 1905 by explaining the Brownian motion of small grains, which had been known for a long time and was directly visible in the microscope, quantitatively by the fact that it originated from randomly clustered collisions of atoms or molecules from the environment. It is only in the last few decades that field ion microscopes and scanning tunneling microscopes, and in recent years also electron microscopes, have made it possible to observe individual atoms directly.
Philosophical considerations
The concept of atomism, namely that matter is made up of basic units - "smallest particles" that cannot be broken down further and further into smaller pieces - has existed for millennia, as has the counter-concept that matter is a continuum that can be divided at will. However, at first these ideas were based exclusively at philosophic considerations and not at empiric experimental investigations. In the process, various properties were attributed to atoms, and very different ones depending on the age, culture and philosophical school.
An early mention of the atom concept in philosophy is known from India. The Nyaya and Vaisheshika schools developed elaborate theories of how atoms coalesced into more complex entities (first in pairs, then three pairs each).
In Greek philosophy, the concept of the atom is first recorded in the 5th century BC by Leucippus. His student Democritus systematized it and introduced the term átomos (ἄτομος), which roughly means "the indivisible", i.e. an object that cannot be further divided. This term was adopted at the end of the 18th century for the then hypothetical smallest units of the chemical elements of incipient modern chemistry, for atoms cannot indeed be "cut up" by chemical methods.
Experimental scientists adopted the hypothesis of the atom at the end of the 18th century, because this hypothesis offered an elegant explanation for new discoveries in chemistry within the framework of a particle model of matter. But at the same time, the opposite idea, that matter was a continuum, was maintained by philosophers and also among natural scientists into the 20th century.
Scientific exploration
See also: List of atomic models
In the course of scientific research, the existence of atoms has been confirmed. Many different atomic models were developed to describe their structure. Especially the hydrogen atom as the simplest of all atoms was important. Some of the models are no longer used today and are only of interest in the history of science. Others, depending on the field of application, are still considered to be useful approximations today. As a rule, the simplest model is taken, which is still sufficient in the given context to clarify the questions that arise.
Many of the discoveries mentioned below (if after 1900) were awarded the Nobel Prize in Physics or Chemistry.
Confirmation of the atomic hypothesis
In his 1661 work The Sceptical Chymist, Robert Boyle argued that matter was made up of various combinations of different corpuscules and not of the four elements of alchemy: water, earth, fire, air. He thus prepared the way for the overcoming of alchemy by the elemental and atomic concept of modern chemistry.
In 1740, Daniel Bernoulli showed that the uniform pressure of gases on the walls of containers, in particular Boyle and Mariotte's law, can be explained by countless collisions of minute particles. His research thus became the forerunner of the kinetic theory of gases and statisticalmechanics.
From the end of the 18th century, the notion of atoms was used to attribute the well-defined angles at the edges and corners of gemstones to the various possible stratifications of hard spheres.
After Antoine Lavoisier had coined today's concept of the chemical element in 1789 and correctly identified the first elements, John Dalton used the atomic concept in 1803 to explain why elements always react with each other in proportions of small whole numbers (law of multiple proportions). He assumed that every element consists of atoms of the same kind, which can combine with each other according to fixed rules and thus form substances with different material properties. He also assumed that all the atoms of an element had the same mass, and thus founded the concept of atomic weight.
In 1811, Amedeo Avogadro was able to summarize the observations on the chemical and physical behavior of gases to the effect that two ideal gases always consist of the same number of identical particles ("molecules") at the same values of volume, pressure and temperature of the gas. In the case of elementary gases such as hydrogen, oxygen or nitrogen, the molecules always consist of two atoms of the element (Avogadro's law).
In 1866, Johann Loschmidt was able to determine the size of air molecules by using a formula derived by James C. Maxwell from the kinetic theory of gases to evaluate the values for internal friction in air measured by George Stokes. This enabled him to determine the weight of a molecule of air. He also obtained the Loschmidt number named after him as the number of air molecules per cubic centimeter (under normal conditions).
As a result of the work of Avogadro and Stanislao Cannizzaro, it was assumed that atoms do not occur as single particles, but only as components of molecules consisting of at least two atoms. However, in 1876 August Kundt and Emil Warburg made the first demonstration of a monatomic gas. They determined the adiabatic exponent of mercury vapour at high temperature and obtained a value which, according to the kinetic theory of gases, can only occur for particles in the form of real mass points. From 1895 onwards, corresponding observations were made on the newly discovered noble gases.
In 1905, after the publication of his dissertation on the determination of molecular dimensions, Albert Einstein proposed an experiment to quantitatively test the hypothesis of the existence of atoms by means of the trembling motion of small particles in water. According to his theory, due to the irregularity of the collisions by the water molecules, the particles would have to perform small movements that were nevertheless visible under the microscope. Einstein was initially unaware that he had used this theory to quantitatively explain the Brownian motion of pollen, which had been known since 1827 and for whose cause Christian Wiener had first assumed molecular collisions in 1863. According to Einstein's formulas, the strength of the trembling motion depends on the mass of the colliding molecules, and on this basis the French physicist Jean Perrin determined the molecular mass experimentally and found similar results to Loschmidt. This work contributed decisively to the general acceptance of what had until then been called the "atomic hypothesis".
Divisibility and structure of atoms
Joseph John Thomson discovered in 1897 that the cathode rays consist of particles of definite charge and mass, and that their mass is less than one thousandth of the mass of the atom. These particles were called electrons and proved to be a constituent of all matter, contradicting the concept of the atom as an indivisible entity. Thomson believed that the electrons gave the atom its mass and that they were distributed in the atom in a massless, positively charged medium like "raisins in a cake" (Thomson's atomic model).
Radioactivity, discovered shortly before, was associated with transformations of different types of atoms into each other by Ernest Rutherford and Frederick Soddy in 1903. In 1908, they were able to demonstrate that the α-particles, which form the alpha radiation, become helium atoms.
Together with his research group, Ernest Rutherford bombarded a gold foil with α-particles in 1909. He found that most of the particles penetrated the foil almost unhindered, but a few were deflected by much larger angles than would be possible according to Thomson's model. Rutherford concluded that almost the entire mass of the atom was concentrated in a much smaller, electrically charged volume in the center of the atom, thus creating the Rutherford model of the atom, which has been valid ever since, with the basic structure of the atom consisting of an atomic nucleus and an atomic shell. The highly deflected α-particles were those that happened to come closer to a nucleus than about one hundredth of the atomic radius. The charge number of the atomic nucleus turned out to be the chemical atomic number of the element in question, and α-particles turned out to be the atomic nuclei of helium.
In 1911, the chemist Frederick Soddy determined that some of the natural radioactive elements had to consist of atoms with different masses and different radioactivity. The term isotope for physically different atoms of the same chemical element was proposed by Margaret Todd in 1913. Since isotopes of the same element could not be distinguished by their chemical behavior, the physicist J.J. Thomson developed the first mass spectrometer to physically separate them. Using neon as an example, he was thus able to demonstrate in 1913 that there are also stable elements with several isotopes.
In 1918, using a mass spectrometer of considerably greater accuracy, Francis William Aston found that almost all elements are mixtures of several isotopes, the masses of the individual isotopes always being (nearly) integer multiples of the mass of the hydrogen atom. In 1919, Rutherford demonstrated in the first observed nuclear reaction that by bombarding the nuclei of nitrogen atoms with α-particles, the nuclei of hydrogen atoms can be shot out. To these he gave the name proton and developed a model of the atom in which atoms consist only of protons and electrons, the protons and some of the electrons forming the small, heavy atomic nucleus, the remaining electrons forming the large, light atomic shell. However, the idea of electrons in the atomic nucleus turned out to be problematic and was finally dropped in 1932 after the neutron was demonstrated by James Chadwick to be a neutral nuclear building block with about the same mass as the proton. This gave rise to today's atomic model: the atomic nucleus is composed of as many protons as the atomic number indicates, plus as many neutrons as necessary to achieve the isotopic mass in question; the atomic shell consists of as many electrons as necessary to make the whole atom neutral.
Structure of the atomic shell
→ Main article: Atomic shell
The observed properties (such as size, stability, reaction modes) of the atomic shell could not find an explanation within the framework of classical physics. In 1913, Niels Bohr was able to explain how the spectral lines in the optical spectra of pure elements, which are absolutely characteristic for the respective element, are formed (spectral analysis according to Robert Wilhelm Bunsen and Gustav Robert Kirchhoff 1859) by using new quantization rules with the help of Planck's quantum of action. In the Franck-Hertz experiment, the quantized energy absorption and emission of mercury atoms could be experimentally confirmed. Although Bohr's atomic model was only valid for systems with only one electron (at that time only hydrogen and ionized helium), it formed the basis for a series of refinements in the course of the following decade. In the shell model, they led to a first understanding of the structure of the electron shells of all elements and thus also to a physical understanding of the chemical periodic table. Thus Bohr's atomic model became the basis of the popular image of the atom as a small planetary system.
In 1925 Werner Heisenberg, together with Max Born, Pascual Jordan, Wolfgang Pauli and others, developed matrix mechanics. In 1926, Erwin Schrödinger replaced the quantization rules with his wave mechanics. It describes electrons not as mass points on certain plane orbits, but as standing matter waves extended in three dimensions. Both forms of a new "quantum mechanics" could correctly explain the spectrum of the hydrogen atom. As a consequence of these descriptions, it is, among other things, inadmissible to ascribe exact values for location and momentum to an electron at the same time. This fact was formulated by Heisenberg in 1927 in the uncertainty principle. According to this, instead of the motion on certain orbits, only probability distributions for ranges of values of location and momentum can be given, an idea that is difficult to illustrate. The quantized orbits of the Bohr model correspond here to "atomic orbitals". Among other things, they indicate how the residence probability of the electrons is concentrated in the vicinity of the atomic nucleus, and thus determine the real size of the atom.
The description of the properties of atoms succeeded much better with these first fully quantum mechanical atomic models than with the precursor models. In particular, the spectral lines and the structure of the atomic shell in spatial and energetic terms could be represented even for atoms with several electrons, including the exact possibilities of forming bound states with the atomic shells of other atoms, i.e. stable molecules. Therefore, the Bohr model of the atom was discarded in favor of the quantum mechanical orbital model of the atom.
The orbital model is until today the basis and starting point of exact quantum mechanical calculations of almost all properties of atoms. The orbital model in the case of an atom with more than one electron can be described physically as an approximation, namely as a one-particle approximation that attributes a specific orbital to each individual electron. A state formed in this way is called the configuration of the atom and belongs to the simplest type of multi-particle states in quantum mechanics. More accurate models take into account that, according to the rules of quantum mechanics, the shell can also be in a state formed by superposition of different configurations, that is, where different electron configurations exist simultaneously with different probability amplitudes (configuration mixing). Hereby the most exact calculations of energy levels and interactions of atoms become possible. However, because of the mathematical effort required, simpler atomic models continue to be used where possible. In addition to the shell model, the Thomas-Fermi model should be mentioned here, in which the electron shell is treated as an ideal electron gas ("Fermi gas") bound in the potential well, the density of which, together with the nuclear charge, determines the shape of the electrostatic potential well.
Structure of the atomic nucleus
→ Main article: Atomic nucleus
For the discovery of the atomic nucleus and its composition of protons and neutrons, see the section "Divisibility and structure of atoms" above. Here follow keywords for research of further properties of nuclei.
Binding energy
The binding energy of nucleons is the cause of the high energy of the quanta of radioactive radiation. It exceeds the chemical binding energy of molecules by five to six orders of magnitude. From 1935 onwards, a rough model was successful for the first time, the droplet model of C.F. von Weizsäcker and Hans Bethe. With this, for nuclei of about 10 nucleons and more, the initial increase of the average binding energy up to about was explained by the increasing number in which the nucleons bind with their respective neighbours due to the actual nuclear forces, and thereafter the decrease of the average binding energy due to the increasing electrostatic repulsion affecting all protons among themselves.
Nuclear fusion and fission
See also: The discovery of nuclear fission
Since the maximum of the average binding energy is at medium-heavy nuclei, it means energy release both when very light nuclei fuse and when very heavy nuclei split. Fusion of hydrogen to helium was identified as the energy source of stars in 1938. Fission after neutron capture was first demonstrated in 1938 on primordial nuclei (of the isotope U-235) by Otto Hahn and Fritz Strassmann. Thereafter, nuclear research was considerably intensified, leading to the first atomic bombs in 1945, hydrogen bombs in 1952, and the use of atomic energy for energy supply from the mid-1950s.
Shell model and unified model
Much more detailed than the droplet model is the shell model of nuclei established in 1949 by J.H.D. Jensen and Maria Goeppert-Mayer. Similar to the shell model of atoms, it assumes a particular orbital for each nucleon in a common spherically symmetric potential well. This can be used to explain a wealth of data about the ground states and excited states of nuclei, for example their nuclear spin, magnetic dipole and electric quadrupole moment, and their decay and reaction modes. In the early 1960s, Aage Bohr, Ben Mottelson and James Rainwater succeeded in combining this single-particle model with the aspects of collective motion, which also made the deviations from the spherical shape in certain ranges of nucleon numbers understandable.
Origin of the nuclear forces
In the 1970s, the short-range nuclear forces could be attributed to the strong interaction between quarks.
Structure of proton and neutron
From the 1950s onwards, the development of improved particle accelerators and particle detectors made it possible to study atoms and especially atomic nuclei when they were bombarded with particles of very high energy. At the end of the 1960s, the "deep elastic scattering" of electrons by atomic nuclei showed that neutrons and protons are not indivisible entities either, but are composed of quarks.
Some advanced experiments with atoms
In 1951, Erwin Müller developed the field ion microscope and was able for the first time to produce an image of a needle tip that was magnified in a direct manner to such an extent that individual atoms became visible in it (albeit only as blurred spots). In 1953, Wolfgang Paul developed the magnetic ion trap (Paul trap), in which individual ions could be stored and examined with ever greater accuracy.
In 1985, a research group led by Steven Chu developed laser cooling, a method of greatly reducing the temperature of a collection of atoms by means of laser radiation. In the same year, a group led by William D. Phillips succeeded in trapping neutral sodium atoms in a magneto-optical trap. By combining these techniques with a method using the Doppler effect, a group led by Claude Cohen-Tannoudji succeeded in cooling small amounts of atoms to temperatures of a few microkelvin. This method allows atoms to be studied with the highest precision; it also enabled the experimental realisation of Bose-Einstein condensation.
In the early 1980s, Gerd Binnig and Heinrich Rohrer developed the scanning tunneling microscope, in which a needle tip scans a surface so finely by means of the tunnel effect that individual atoms become visible. This also made it possible to place atoms individually in specific locations. In the 1990s, Serge Haroche and David Wineland were able to successfully study the interaction of a single atom with a single photon in experiments. In the 2000s, the manageability of single atoms was used, among other things, to produce a transistor from just one metal atom with organic ligands.
Since the late 1980s, Rydberg atoms have been created by multiple excitation with a laser pulse. In a Rydberg atom, an electron is excited to such a high energy state that it orbits the atomic nucleus, and in some cases also the entire atomic body, consisting of the atomic nucleus and the remaining electrons, at a great distance and its behaviour thus approaches that of a classical particle. Rydberg atoms can be over 100,000 times larger than unexcited atoms. Since they are extremely sensitive to external fields, they can be used, for example, to study in detail the interaction of a single atom with a single photon. If two or more electrons are excited in such states, they are called planetary atoms.
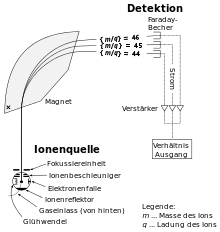

Simple mass spectrometer (schematic diagram)
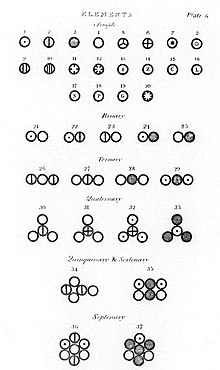

Various atoms and molecules as illustrated in A New System of Chemical Philosophy (1808) by John Dalton.
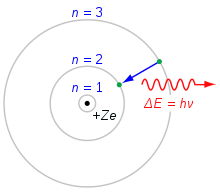

Illustration of Bohr's model of the hydrogen atom (Z=1) with an electron jumping between fixed orbits, emitting a photon with a certain frequency f.
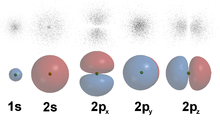

Orbital model of the atom: representation of the atomic orbitals of the first (2 electrons) and second (8 electrons) electron shells
Classification
Elements, isotopes, nuclides
The differentiation and designation of various types of atoms is initially based on the structure of the atomic nucleus, while the state of the shell is indicated by additional symbols if necessary. Key figures are the proton number (atomic number, nuclear charge number) Z, the neutron number N of the nucleus, and the mass number A=Z+N formed from this. Depending on their proton number, the atoms belong to one of the 118 known chemical elements, from hydrogen with Z=1 to oganesson with Z=118. 91 of them have been discovered in natural occurrences, 27 only after artificial production by nuclear reactions. The order of the elements is graphically illustrated in the periodic table - important for chemistry. In it, the elements are arranged in ascending atomic number in the form of a table. Each row is called a period of the periodic table and ends when the respective orbital is fully occupied with electrons (noble gas). In the next rows, the chemical character of the elements is repeated due to the stepwise electron occupation of the next orbitals. Thus, elements with similar chemical properties stand in a column below each other; they form a group of the periodic table.
Atoms of an element that differ in neutron number belong to different isotopes of the element. In total, the 118 elements consist of about 2800 isotopes, of which 2500 have been artificially produced. Isotopes, with the exceptions of the hydrogen isotopes deuterium and tritium, are named after the chemical element and the mass number. The symbol for a particular isotope of element is of the form
,
or X-A (examples:
,
, Pb-208). The specification of the proton number Z is redundant, since it is already
given by the atomic number of the element
Nuclide is the very general term for atomic species, whether they belong to the same element or not. The nuclide map or isotope map - important for nuclear physics and its applications - is a table in which each type of atom is given its own place. This is done by plotting the number of protons on one axis and the number of neutrons on the other. Often the stability and, in the case of unstable nuclides, also the type of transformation or the magnitude of the half-life are represented by certain colours and, if necessary, also division of the place assigned to the isotope.
Stable and unstable (radioactive) atoms
The nucleus of a nuclide can exist either in the energetic ground state or in one of the various excited states. If among them are relatively long-lived, so-called metastable states, these are called isomers and are counted as separate nuclides (symbol
,
or similar). According to this definition, a total of about 3200 nuclides are known as of 2003.
In nuclear physics, nuclides with different proton numbers but the same mass number are called isobars. More rarely, nuclides with different proton numbers but the same neutron number are grouped under the name isotones.
Only about 250 isotopes of 80 elements have a stable nucleus. All other atoms are unstable and sooner or later transform into atoms of a stable isotope. Since they generally emit ionizing radiation in the process, they are also called radioisotopes or radionuclides. On Earth, in addition to all 250 stable isotopes, 30 radioisotopes have been found in natural deposits, distributed among 10 radioactive elements that cause natural radioactivity. Many more short-lived isotopes exist inside stars, especially during the supernova phase.
Rare and theoretical forms
A Rydberg atom is an atom in which an electron is excited to such a high energy state that it orbits the atomic nucleus, and in some cases the entire atomic body consisting of the atomic nucleus and the remaining electrons, at a great distance and its behaviour thus resembles that of a classical particle. Rydberg atoms can be over 100,000 times larger than unexcited atoms. Since they are extremely sensitive to external fields, they can be used, for example, to study the interaction with a single photon in detail. If two or more electrons are excited in such states, they are called planetary atoms.
In a partly figurative sense, exotic atoms are also those systems which, from a physical point of view, show certain similarities to ordinary atoms. In them, for example, one of the protons, neutrons or electrons may have been replaced by another particle of the same charge. For example, if an electron is replaced by a heavier muon, a muonic atom is formed. An exotic atom in which an electron is bound to a positron, the positively charged antiparticle of the electron, instead of to a proton is called a positronium. Atoms composed entirely of antiparticles to normal matter are also possible. For example, anti-hydrogen atoms were first artificially produced and detected at CERN in Geneva in 1995. Such exotic atoms can be used, among other things, to test fundamental physical theories about the symmetry between particles and antiparticles.
Furthermore, the name atom is sometimes used for two-particle systems that are not held together by electromagnetic interaction but by the strong interaction. Such a quarkonium is a short-lived elementary particle of the meson type, which is composed of a quark and an antiquark. A quarkonium atom can be classified in its various metastable states by quantum numbers in the same way as the hydrogen atom.
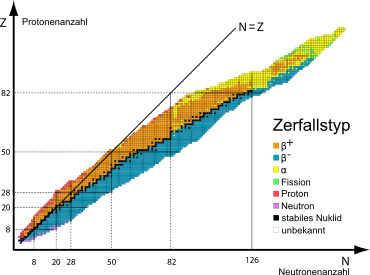

Nuclide Map
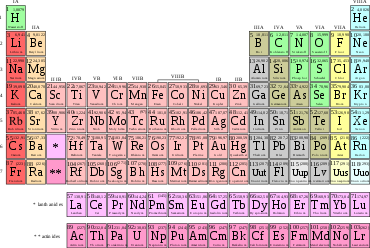

Periodic table
Questions and Answers
Q: What is an atom?
A: An atom is the most basic unit of matter. It is the smallest unit of matter that can combine with other atoms to make molecules and more complex matter that have specific chemical qualities.
Q: How big are atoms?
A: Atoms are very small, ranging from 0.1 to 0.5 nanometers across, which is about 100,000 times smaller than the width of a human hair.
Q: What are the three types of subatomic particles that make up an atom?
A: The three types of subatomic particles that make up an atom are protons, neutrons, and electrons. Protons and neutrons are heavier and located in the nucleus while electrons are lighter weight and attracted to the nucleus by electromagnetic force due to their opposite electric charges.
Q: How many elements occur naturally in the world?
A: About 92 elements occur naturally in the world.
Q: What happens when an atom has more or less electrons than protons?
A: If an atom has more or less electrons than protons it is called an ion, and has an electric charge.
Q: What type of changes happen if forces inside atoms become too weak to hold them together?
A: If forces inside atoms become too weak to hold them together they can be changed into another type of atom or destroyed altogether; this process is studied in nuclear physics.