Neuron
Neuron and neuronal are redirections to this article. For other meanings, see Neuron (disambiguation).
A nerve cell, also called a neuron (from the ancient Greek νεῦρον neũron, German 'Flechse', 'Sehne'; 'nerve'), is a cell specialized in the conduction and transmission of excitation, which occurs as a cell type in tissue animals and thus in almost all multicellular animals. The totality of all the nerve cells of an animal, together with the glial cells, forms the nervous system.
A typical mammalian neuron has a cell body and cell processes of two types: the dendrites and the neurite or axon. The branched dendrites primarily receive excitation from other cells. The neurite of a neuron, which is surrounded by glial cells, can be more than a meter long and initially serves to transmit an excitation of this cell to the vicinity of other cells. In this process, a voltage change is transmitted across the process by allowing brief ionic currents through special channels in the cell membrane.
The axon ends are in contact with other nerve cells, muscle cells (neuromuscular end plate) or glandular cells via synapses, at which the excitation is rarely passed on directly electrically, but is usually transmitted chemically by means of messenger substances (neurotransmitters). Some nerve cells can also release signalling substances into the bloodstream, e.g. modified neurons in the adrenal medulla or in the hypothalamus as secretion of neurohormones.
It is estimated that the human brain, with a mass of one and a half kilograms, consists of almost ninety billion nerve cells and a similar number of glial cells.
The nerve cell is the basic structural and functional unit of the nervous system. Its designation as a neuron goes back to Heinrich Wilhelm Waldeyer (1881).
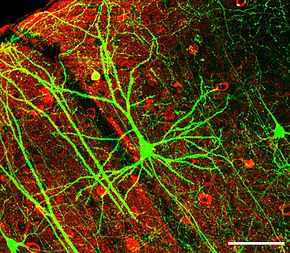

Microscopic image of the cerebral cortex of a mouse. The pyramidal cell with a large dendritic tree in the center of the image expresses green fluorescent protein. GABA-producing interneurons can be seen in red. (Length of the scale at the bottom right: 100 µm)
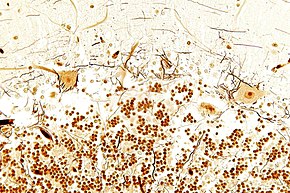

Tissue section from the cerebellar cortex (Bielschowsky stain): Surrounded by glia, the top image shows the light brown stained processes and large cell bodies of a few Purkinje cells in close contact with some black stained processes of basket cells; below, the location of cerebellar granule cells, which account for more than half of all neurons in mammals.
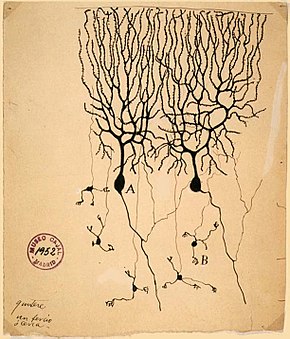

Two Purkinje cells and five granule cells from the cerebellum of a pigeon (drawn by Santiago Ramón y Cajal, 1899).
Structure
nerve cell structure |
Dendrite Cell body Axon Nucleus Ranvier lacing ring Axon terminals Schwann cell Myelin sheath |
The cell body
→ Main article: Perikaryon
Each nerve cell has a body, which is called soma or neuroanatomically perikaryon, as in other cells. Here, the perikaryon includes the plasmatic area around the nucleus, excluding cell processes such as neurite and dendrites. In addition to the nucleus, the cell body contains various organelles such as rough and smooth endoplasmic reticulum, mitochondria, the Golgi apparatus and others. Characteristic for the cell body of neurons is the condensed occurrence of the endoplasmic reticulum and its accumulation as Nissl substance into Nissl clods, which in contrast are absent in the processes and also in the axon hillock. In the soma, all proteins and other important substances are formed that are necessary for the function of the neuron; depending on the type and size of the neuron, its perikaryon measures between 5 µm and more than 100 µm.
Excitations from other cells are transmitted locally to the branched dendrites and spread as electrical voltage changes across the membrane of the nerve cell, becoming weaker with increasing distance. Superimposed on each other, they converge in the perikaryon, where they are collected and processed in an integrating manner. This occurs through spatial and temporal summation of the various changes in the membrane potential.
It depends on the result of the summation at the location of the axon hill whether the threshold potential is exceeded here and thus an action potential is now formed or not (see all-or-nothing law). Emerging action potentials are an expression of the excitation of a nerve cell. They are successively transmitted via the axon process. At its endings, the excitation is transmitted to other cells.
The dendrites
→ Main article: Dendrite (biology)
Various plasmatic processes originate from the cell body of a nerve cell. The dendrites (Greek δένδρον dendron, German 'tree') are finely branched nerve cell processes that grow out from the soma and form contact points for other cells, whose excitation can be transmitted here to the nerve cell. Through a synapse, the neuron is linked to a specific cell and receives signals with the locally associated postsynaptic membrane region of a dendrite. The dendrite tree of a single neuron may have several thousand such synaptic contacts, through which it receives different signals, each of which is mapped locally as specific changes in postsynaptic membrane potential. The individual contact sites can be designed differently in each case; in some neurons, special formations in the form of dendritic spines are found for this purpose. Synaptic activation at the dendrite alone is capable of causing changes that can persist for a long time (see synaptic plasticity).
The axon hillock
→ Main article: Axon hillock
The cone of origin of the neurite or axon hillock, from which the axon of a nerve cell emerges, forms a special area of the cell body that is free of Nissl clods. Here, the threshold potential is significantly lower, so that postsynaptic potentials are most likely to trigger an action potential at this point of the perikaryon. The action potentials formed in the subsequent first segment of the axon, its initial segment, are propagated through the axon. The axon hillock is thus the site where postsynaptic potential changes are integrated and converted into series of action potentials, thus transcoding analog into digital signals. Its low threshold potential and the location of the axon hillock ensure that, in the event of excitation of the nerve cell, action potentials are generated at this site and transmitted via its axon.
The axon
→ Main article: Axon and nerve fiber
The axon (Greek ἄξων axōn, German 'axis') of a nerve cell is the glial neurite originating at the axon hillock, through which its excitation is transmitted to other cells. Action potentials triggered in the initial section are carried on via the axon and its lateral branches (collaterals) to the terminal sections, which usually form a terminal knob as a presynaptic termination. Depending on the location of the target cell and on the type and size of the nerve cell, considerable differences occur in the length and diameter of the axons. The axons of mammalian nerve cells are about 0.05 µm to 20 µm thick and in humans approximately between 1 µm and 1 m long.
In the course, these extensions of the nerve cells are sheathed by glial cells of the nervous system - in the peripheral by the Schwann cells and in the central by the oligodendroglia. Axon and sheath together form a nerve fiber. When glial cells envelop the axon cylinder by multiple wrappings, an insulating medullary or myelin sheath is formed around the axon from their membrane lamellae, leaving a narrow gap at each cell boundary - called a Ranvier laced ring or node (nodus) - between the individual glial cells of successive segments (internodes). This structure characterises nerve fibres with a medullary sheath and enables a faster step-by-step conduction of excitation than in the case of so-called medullary nerve fibres with a simple sheath without a myelin sheath.
In the cytoplasm of the axon (axoplasm), a particularly structured cytoskeleton of neurofibrils and microtubules is found, which serves in particular for axonal transport within this, often extraordinarily long, cell process. The proteins and membrane envelopes synthesized in the soma are transported to the terminal axon via this cytoskeleton (anterograde). However, rapid transport of substances also takes place in the opposite direction towards the cell body (retrograde transport).
The myelin sheath
→ Main article: Myelin sheath
The sheathing of an axon by glial cells with sectional insulating multiple wrappings is called myelin sheath (Greek μυελός myelos, German 'Mark'). Such medullary nerve fibers conduct signals about ten times faster than medullary ones of the same axon diameter, allowing an organism to respond correspondingly faster to stimuli in the environment. While in the central nervous system oligodendrocytes myelinate axons, in the peripheral it is Schwann cells that surround an axon and wrap around it up to fifty times for the myelin sheath. Because the biomembranes of the two types of glial cells differ somewhat, myelin peripherally has a different composition of phospholipids and proteins than centrally.
A single Schwann cell builds up an approximately 1 mm long myelin sheath section for the internodal axon in tightly packed layers consisting almost entirely of lamellae of its cell membrane. One myelinated axon segment (internode) separates from the next at the node (nodus). The myelin sheath is thus formed longitudinally from a series of glial cells and interrupted between adjacent ones at regular intervals by narrow so-called rings of Ranvier's lacing. These play an essential role in the transmission of action potentials along the myelinated axon. Because of the insulating sheath, the voltage changes jump depolarizingly from lacing ring to lacing ring (saltatory excitation conduction), where an action potential is built up, whereas in non-myelinated nerve fibers this happens progressively along the entire length of the axon membrane (axolemma) and thus takes longer. The initial and terminal parts of an axon are usually not myelinated.
The end button
→ Main article: Presynaptic termination
An axon and each of its branches to collaterals ends with a so-called terminal knob, also called terminal bulb or terminal plate or axon terminal, which represents the presynaptic part of a synapse. Synapses link nerve cells to each other or to other cells in such a way that an excitation can be transmitted to individual downstream cells.
The terminal knob on the terminal axon serves as the presynapse of the "transmitter cell". In the area below the presynaptic cell membrane, it contains molecules of a neurotransmitter packaged in separate membrane envelopes of synaptic vesicles. When a conducted action potential reaches the terminal head during excitation, increased calcium ions (Ca2+) flow into the presynaptic terminal through voltage-gated calcium channels. This short-term calcium influx triggers a chain of interactions between particular proteins, as a result of which synaptic vesicles are deposited at the presynaptic cell membrane and fuse here by membrane fusion. The vesicle content with neurotransmitter is thus released into the narrow intercellular space by exocytosis.
On the opposite side of the synaptic cleft is the postsynaptic membrane area of the "recipient cell", which is equipped with receptors to which the transmitter molecules bind. Ion channels are then opened, which leads to a change in the electrical voltage at the postsynaptic membrane, which spreads to neighbouring regions. The synaptic cleft between the two cells is a narrow space of about 30 nm that is part of the extracellular space in which the transmitter molecules are distributed by diffusion.
The synapse
→ Main article: Synapse
Function
The synapse is the site where excitation can be transferred from one cell to another. In most cases, a neurotransmitter is used to bridge the narrow gap between the cells, called the synaptic cleft. Synapses can be thought of as interfaces between cells through which neurons communicate with other cells.
A single neuron can be related to other cells via numerous synapses, both in terms of incoming and outgoing signals. A Purkinje cell of the cerebellum, for example, receives input signals from other neurons via about 100,000 dendritic synapses. A granule cell in the cerebellum sends output signals to other neurons, including Purkinje cells, via several hundred synapses. The total number of synapses in the human brain is estimated to be slightly less than a quadrillion.
The neurons as parts of the nervous system are rarely directly connected by electrical synapses, but mostly by chemical synapses. This transmission of signals by means of messenger substances can be modified or modulated in various ways. The conditions of signal transmission are thus not completely fixed, but can be shaped within certain limits, which is referred to as synaptic plasticity. Interconnected in this way, neurons as a whole form a malleable neuronal network that can change in its effect through the act of use (neuronal plasticity).
In terms of information technology, basic features of the networking of neurons can be reproduced in a highly simplified way. Similarly, an artificial neural network with a different architecture can be designed, which, for example, can be trained step by step in such a way that it is suitable for recognizing complex patterns. Here, the learning process is anchored in shifts in the weights between neuron elements or their threshold value.
Neurotransmitter
→ Main article: Neurotransmitter
Neurotransmitters serve as messengers at chemical synapses for the transmission of excitation from one nerve cell to another (transmission). The transmitters are released presynaptically when the "transmitter cell" is excited, bridge the synaptic gap between the cells and are received postsynaptically by the "receiver cell" by means of special receptor proteins. These specifically recognize the respective transmitter molecule by its spatial shape and charge distribution through complementary structures and bind it reversibly.
The binding leads to a remodelling of the receptor structure (conformational change). This can have a direct (ionotropic) triggering or indirect (metabotropic) knock-on effect on the opening of ion channels in the membrane, thereby briefly permitting ion currents and thus temporarily bringing about a change in the membrane potential of this region. Depending on the configuration of the postsynaptic membrane, a potential difference (postsynaptic potential, PSP) is then evoked as a local response, which either favors or triggers the excitation of the recipient cell (excitatory, excitatory PSP) or impedes or prevents its excitation for a short time (inhibitory, inhibitory PSP).
The excitation transmitted by the transmitter thus has either an excitatory or an inhibitory effect on the downstream cell at a chemical synapse, which is determined by the receptor types and the ion types of the influenced membrane channels in the respective linked cell. In neuromuscular synapses, for example, impulses from excited nerve cells at motor endplates are transmitted to muscle fibers by means of acetylcholine (ACh) as a transmitter substance and have an excitatory effect on these effectors; the postsynaptic endplate potential can thus trigger an action potential of these cells. In the muscle cells this leads to contraction, which can show up at the associated organ of success as shortening of the muscle. The effect of the possibly re-binding transmitter is limited here primarily by its enzymatic decomposition (acetylcholinesterase) in the synaptic cleft.
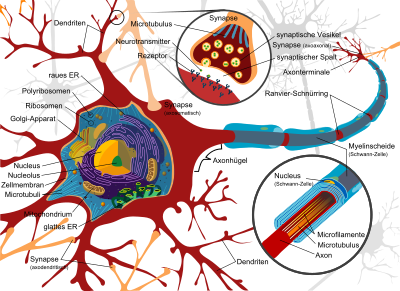

Structure of a neuron: The diagram shows the structure of a vertebrate nerve cell whose axon lies peripherally (in the central nervous system oligodendrocytes form the myelin sheath).
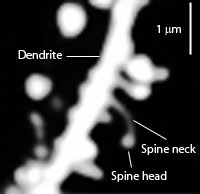

Dendrite with several spines ( dendritic spines )
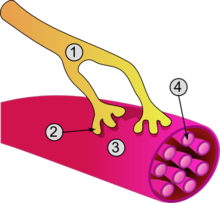

Neuromuscular endplate. 1. axon of a motoneuron2 . Motor end plate3 . Muscle fiber4 . Myofibril
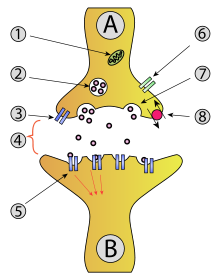

Schematic of excitation transmission at a chemical synapse from nerve cell A with the presynapse to cell B with the postsynapse -. 1 mitochondrion, 2 synaptic vesicle, 3 autoreceptor, 4 synaptic cleft with released unbound neurotransmitters, 5 postsynaptic receptor, 6 calcium channel, 7 exocytosis of vesicle, 8 active transport across cell membrane.
How it works
A nerve cell receives a signal when neurotransmitters that were released by an upstream cell, for example, bind to special receptors in the postsynaptic membrane in the dendrites or the soma of the cell to be excited. Once the excitation has been transferred in this way, it is transmitted via the dendrites to the soma of the nerve cell and from there to the axon hillock. Each of the incoming depolarizations at the various synapses of the nerve cell thereby alters the membrane potential at the axonal membrane, where an action potential is triggered when a threshold is exceeded. In general, the closer a synapse is to the soma, the stronger its influence on the nerve cell; the longer the distance the excitation has to travel, the weaker the influence. Thus, a stronger stimulation of a dendrite results in a stronger depolarization (see second graph in the figure on the right). Stimuli arriving almost simultaneously add up in their effect, which means that an excitation potential builds up within the cell and at the axon hillock (summation).
In the axon hillock, certain factors now decide on the triggering of an action potential according to the rules of the all-or-nothing law, whereby it is decided whether the threshold potential has been reached and exceeded. If this is the case, the release of the action potential along the axon now occurs due to the depolarization of the axon. This in turn occurs at the biomembrane, the so-called axolemma, which demarcates the intracellular area (inside) from the extracellular area (outside).
There are ions inside the axon as well as outside the membrane. The biomembrane of the axon causes different concentrations of ions to exist between the inside and outside, so that a different electrical charge is applied to the outer wall of the axon than to the inside - the cell interior is negatively charged. This is called polarization or resting potential. The axolem performs the production and maintenance of polarization with the help of a sodium-potassium pump, named after the ions of the elements sodium and potassium, which play an important role in the transmission of excitation. This process is called active transport because it requires the supply of energy. If an action potential now travels along the axon to the terminal button due to the change in the concentration gradient of the ions within the axon, this electrical impulse reaches a limit at the end of the axon, since transmission of the electrical signal through the synaptic cleft between the two cells is not possible. The stimulus is passed on chemically via the synapses and transferred to another cell in analogy to the process already described.
Once an action potential has been triggered, the cell needs time to rebuild the membrane potential (repolarization). During this pause, also called the refractory phase, no new action potential can be triggered. Thus, if one of the successive incoming stimuli is so strong that the cell forms an action potential and the subsequent stimulus arrives during the refractory period, the cell does not form a new action potential for it.
The more action potentials the cell fires per unit time, the stronger the stimulus. The conduction of excitation is basically possible in both directions. Due to the inactivation of the sodium channels, the action potentials are preferentially transmitted in one direction. One also says that the nerve cell fires. It can do this up to 500 times in one second.
The prerequisite for the function of the neuron is therefore its ability to receive and transmit an electrical impulse. Important factors play a role in this: electrical excitability (receiving the impulse), resting potential (the ability to integrate it), action potential (relaying and transmitting it) and excitation conduction (transmitting it purposefully).
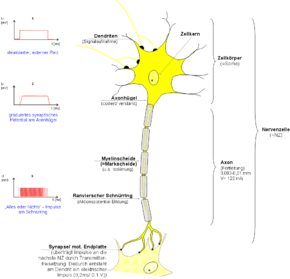

Pulse conduction at a myelinated nerve cell.
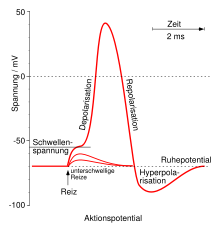

Course of the action potential
Questions and Answers
Q: What is a neuron?
A: A neuron is a nerve cell that carries electrical impulses and is the basic unit of the nervous system.
Q: What are the components of a neuron?
A: A neuron has a cell body (soma or cyton), dendrites, and an axon. Dendrites and axons are nerve fibers.
Q: How many neurons are in the human brain?
A: There are approximately 86 billion neurons in the human brain, with almost all brain cells being neurons.
Q: How many neurons are in the cerebral cortex of the human brain?
A: The human brain has about 16 billion neurons in the cerebral cortex.
Q: What supports the neurons in the brain?
A: Neurons are supported by glial cells and astrocytes.
Q: How do neurons communicate with each other?
A: Neurons are connected to one another through tiny gaps called synapses, which can be chemical or electrical synapses that pass the signal from one neuron to the next.
Q: What is the function of a neuron?
A: The function of a neuron is to convey information throughout the body through electrical and chemical impulses.