Action potential
In physiology, an action potential, abbreviated AP, is a temporary characteristic deviation of the membrane potential of a cell from the resting potential. An action potential forms automatically with a cell-typical course when the cell is excited (excitation) and spreads as an electrical signal across the cell membrane. Colloquially, the action potentials of nerve cells are also called "nerve impulse".
Only excitable cells can form action potentials in response to stimuli or signals, through short-term changes in membrane conductivity as a result of interactions of special voltage-gated ion channels in their membrane. Their time-dependent different activation leads to different ionic currents with correspondingly shifted potential differences. This results in an action potential curve in which the phase of depolarization is followed, after a possible plateau, by the phase of repolarization, with oscillating hyperpolarization. This process runs automatically in typical form when a certain threshold potential is exceeded, and can only be triggered again after a certain refractory period.
In animals, excitable cells include muscle cells and some secretory cells in addition to their nerve cells. Nerve cells receive stimuli or signals from other cells, convert them into membrane potential changes and can form action potentials (excitation formation) as a cell's own signal, which is carried along the axon in a nerve fibre (→ excitation conduction) and transmitted to other cells via synapses (→ excitation transmission). Neuromuscular synapses are used to reach muscle cells, which can also be excited and then form action potentials that are conducted via membrane invaginations to cause contraction of the muscle fiber. Glandular cells are reached via neuroglandular synapses; special neuroendocrine cells can form action potentials which are followed by a release of neurohormones.
In addition, action potentials also occur in unicellular organisms - for example in slipper animals and diatoms - as well as in multicellular algae (stoneworts), vascular plants (mimosa) and fungi.
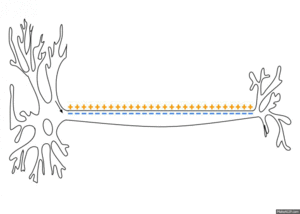

Conduction of an action potential across the membrane along the axon of a neuron.
History
The phenomenon of muscle movements as a result of electrical forces was discovered by the Bolognese Luigi Aloisio Galvani as the twitching of thighs of dissected frogs. His findings of an "animal electrical fluid" in nerves and muscles, published in 1791, prompted Alessandro Volta to conduct his research on galvanism, which led to the invention of the first battery in 1799 - called Volta's column. Volta also used this to study the effect of DC voltage on living things.
In 1952, Alan Lloyd Hodgkin and Andrew Fielding Huxley presented a mathematical model that explained the emergence of the action potential in the giant axon of the squid through the interplay of different ion channels and became famous under the name Hodgkin-Huxley model. For this discovery, the two researchers, together with John Eccles, received the Nobel Prize for Medicine in 1963.
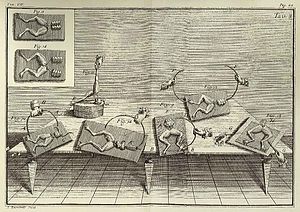

Galvani experimented on frogs' legs (De viribus electricitatis in motu musculari, plate 3).
Basics
An action potential takes a form that is typical for the cell type. In nerve cells it often lasts only about one to two milliseconds, in skeletal muscle cells hardly longer, in cardiac muscle cells usually more than 200 ms. There are no stimulus-dependent stronger or weaker action potentials, but rather all-or-nothing responses. The signal strength therefore results from the frequency of action potentials. In nerve cells, they typically arise at the axon hillock and are propagated in series along the axon. Action potentials can also propagate backward across the cell body and dendrites; the function of this propagation is still under investigation. The axonal propagation from the cell body to the terminal knob is called orthodromic, the opposite antidromic.
Prerequisite for the formation of an action potential are special properties of the plasma membrane of the cell. The specific equipment with different groups of ion channels is reflected in characteristics of the course form. Excitation occurs when the membrane potential shifts away from the resting value and towards less negative values. When this initial pre-depolarization reaches a certain threshold, the so-called threshold potential (approximately at -55 mV), voltage-gated ion channels are activated, which open in a chained sequence, thus enabling ion currents, and inactivate again.
During this chain of opening and closing processes of the channels, the membrane conductivities for different ions thus change temporarily. The associated brief ion currents together lead to a characteristic potential curve. The shape of this potential is the same for each cell, independent of the strength of the suprathreshold stimulus. The short-term changes in the potential now spread (electrotonically) to the neighbouring membrane area and can then excitably lead to the action potential here again, which is the basis of excitation conduction.
Questions and Answers
Q: What is a nerve impulse?
A: A nerve impulse is a series of electrical signals generated in neurons (nerve cells) in response to a stimulus.
Q: What type of cells generate nerve impulses?
A: Nerve impulses are generated in neurons, or nerve cells.
Q: How do nerve impulses respond to stimuli?
A: Nerve impulses are generated in response to external stimuli.
Q: What type of signal is produced by a nerve impulse?
A: A nerve impulse produces an electrical signal.
Q: Where does the electrical signal travel during a nerve impulse?
A: The electrical signal produced by the nerve impulse travels along the neuron.
Q: Is there any other name for neurons?
A: Neurons are also referred to as "nerve cells".