Methane clathrate
Methane hydrate (also called methane clathrate or clathrate hydrate (from Latin clatratus 'latticed'), methane ice or combustible ice) is a naturally occurring, ice-like substance. It is composed of water molecules that form a cage structure via hydrogen bonds in which methane molecules are trapped. The trapped methane originates mainly from microbial methanogenesis and partly from geological processes. As a highly concentrated form of methane that sequesters large amounts of carbon, methane hydrate is a significant component of the global carbon cycle.
Towards the end of the 19th century, the French physicist and chemist Paul Villard investigated the synthesis of methane hydrate and its physicochemical properties. It was initially considered a laboratory curiosity until the 1930s, when it was identified as the cause of natural gas pipeline blockages.
Methane hydrate was first discovered in nature in the 1960s. It is found worldwide in sediment along continental margins, on polar shelves, in the deep sea, and within and below permafrost regions. Although the exact extent of the deposits is unknown, methane hydrate, which has the highest energy density of all naturally occurring forms of methane, is believed to represent one of the largest untapped energy reservoirs on Earth. Estimates of the size of the deposit vary considerably, and the amount of sequestered carbon may exceed the sum of all other fossil fuels. The development of technical applications based on methane hydrate is still in its infancy and includes gas separation, water desalination and space exploration.
Methane hydrate, as a source of atmospheric methane, is thought to have influenced the Earth's historical climate. Disintegration of methane hydrate due to increased environmental temperatures could release methane, a greenhouse gas, on an enormous scale and thus accelerate human-induced climate change.
As part of the marine sediments, it stabilizes them; the role of methane hydrate decay in submarine landslides and landslides is not conclusively understood. Methane hydrate fields at the bottom of the Gulf of Mexico serve as a habitat for ice worms. Their presumed food source is bacteria that live on the methane hydrate and feed on methane.
The possible occurrence of methane hydrate on Mars, Pluto, the moons of Saturn and Jupiter, transneptunian objects, comets and asteroids is the subject of manifold scientific investigations. Methane hydrate may be the source of Titan's methane atmosphere. Suspected methane hydrate deposits on Mars could be important for Mars colonization.
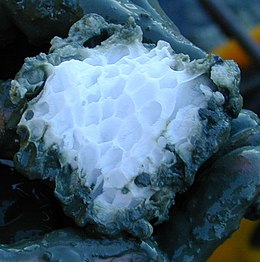

Methane hydrate with honeycomb-like structure from "Hydrate Ridge" off Oregon, USA.
Classification and nomenclature
Methane hydrate belongs to the group of inclusion compounds. These are chemical substances in which a host component forms a cavity in the form of tunnels, channels or layers in which other atoms or molecules are incorporated as a guest component. A subgroup of inclusion compounds are the clathrates or cage compounds. IUPAC defines clathrates as "inclusion compounds in which the guest molecule is in a cage formed by the host molecule or by a lattice of host molecules." (IUPAC: Gold Book) There is no covalent or ionic bonding between guest and host, the attraction is due to van der Waals forces.
Gas hydrates or clathrate hydrates, often just called hydrates, are non-stoichiometric compounds in which water molecules form the host cages in whose cavities small gas atoms or molecules are trapped. If the trapped molecule is methane, the gas hydrate is called methane hydrate.
Methane hydrate exists in three different structures called methane hydrate I, II and H. Structure I is the most common form of naturally occurring methane hydrate. The host cage structure is described using the notation AB, where A is the number of sides per cage face and B is the number of face types that make up a particular cage. In methane hydrate I, water molecules form pentagondodecahedral cages consisting of 12 pentagonal faces with common edges, referred to as 512-cages, and slightly larger cages consisting of hexagonal and pentagonal faces, referred to as 51262-cages. The ratio of 512-cages to 51262-cages is about 1 : 3. In methane hydrate II, the water molecules form 512- and 51264-cages. This structure, called hexakaidecahedron, is formed by guest molecules larger than methane.
Methane hydrate of structure I, which was formed from methane of biogenic origin, contains almost exclusively methane (> 99 %) as a trapped gas component. This form accounts for over 95 % of all methane hydrate deposits. In methane hydrate of structure II, which is of thermogenic origin, other hydrocarbons such as propane are present in addition to methane. Structure H contains even larger molecules such as hexane, methylcyclopentane, cyclohexane and methylcyclohexane. Methane is nevertheless the predominant component in naturally occurring methane hydrate in both structures.
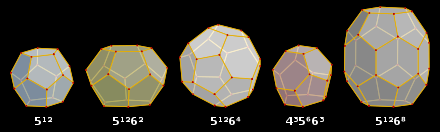

Water cages forming the sI, sII and sH structures of gas hydrates
History
First observations
The first observation of a clathrate hydrate probably dates back to Joseph Priestley, who in 1778 observed the formation of crystals when he passed sulfur dioxide through water at about 0 °C.
"I had observed that with respect to marine acid air and alkaline air that they dissolve ice, and that water impregnated with them is incapable of freezing, at least in such degree of cold as I had exposed them to. The same I find, is the case with fluoric acid air, but it is not so at all with vitriolic acid air, which, entirely contrary to my expectation, I find to be altogether different. [...] But whereas water impregnated with fixed air discharges it when it is converted into ice, water impregnated with vitriolic acid air, and then frozen retains it as strongly as ever."
"I had observed in regard to marine acid air (hydrogen chloride) and alkaline air (ammonia) that they dissolve ice, and that water impregnated with them is incapable of freezing, at least at such a degree of cold as I had exposed it to. The same, I find, is the case with fluoric acid air (tetrafluorosilane), but it is not at all so with vitriolic acid air (sulphur dioxide), which I find, quite contrary to my expectation, to be quite different. [...] But whereas water impregnated with fixed air (carbon dioxide) gives it off when converted into ice, water impregnated with vitriolic acid air, and then frozen, retains it as strongly as ever."
- Joseph Priestley
Humphry Davy noticed a similar phenomenon in 1810 when he cooled water mixed with chlorine to 9 °C. Michael Faraday determined the chemical composition of the hydrate to be one mole of chlorine to ten moles of water, although the actual composition is closer to one mole of chlorine to eight moles of water. In the first half of the 19th century, other chemists devoted themselves to the study of gas hydrates. For example, the chemist Carl Löwig synthesized bromine hydrate in 1829, and Friedrich Wöhler discovered the hydrate of hydrogen sulfide in 1840. The first synthesis of carbon dioxide hydrate was achieved by Zygmunt Wróblewski in 1882. Around 1884, Bakhuis Roozeboom investigated further hydrates, including the hydrate of sulfur dioxide already described by Priestley.
Discovery of the methane hydrate
From 1888, Paul Villard studied the hydrates of hydrocarbons. In that year he discovered methane hydrate, the hydrates of ethane, ethene, ethyne, and nitrous oxide, and in 1890 the hydrate of propane. Methane hydrate is produced at low temperatures under pressure with an excess of the gas; excess methane is removed by depressurization. Villard established the rule according to which the general composition of gas hydrates are described by the formula M + 6 H2O; the rule applies approximately to small molecules occurring in structure I.
In collaboration with Villard, Robert Hippolyte de Forcrand synthesized the hydrates of chloromethane, as well as mixed gas hydrates. He succeeded in preparing the hydrates of inert gases such as argon in 1896, of krypton in 1923, and of xenon in 1925. Furthermore, the existence of double hydrates containing molecules of two hydrate-forming substances was discovered.
The focus of scientific work at this time was on the identification of compounds that formed hydrates and their quantitative composition. Although other scientists turned to the study of hydrates and researched their properties and chemical composition, the field of hydrate research did not initially arouse industrial interest.
Blockage of pipelines
This changed in the 1930s, when natural gas production and transport in pipelines under high pressure gained economic importance. In certain sections of natural gas pipelines, the Joule-Thomson effect caused the temperature to drop sharply. In these sections, an ice-like substance was found clogging the pipelines. Hammerschmidt proved in 1934 that methane formed methane hydrate with the water present in the natural gas stream, and that this clogged the pipelines, not ice as originally thought. His discovery triggered a new phase of methane hydrate research, as methane hydrate plugs in natural gas pipelines were problematic for the natural gas industry, causing economic losses and environmental risks.
The associated problems and accidents led to a wide range of research activities aimed at preventing the formation of methane hydrate when handling natural gas and crude oil. They included research into additives that dissolve methane hydrate or inhibit its formation. Investigation of its thermodynamic stability limits and the kinetics of formation and dissolution allowed prediction of methane hydrate blockage formation in gas pipelines. The oil and gas industry intensified the research again after it began to extract oil deposits in the deep sea, where the necessary conditions for methane hydrate formation prevail.
As early as the mid-1930s, it was suspected that methane hydrate was a clathrate compound. This was confirmed in the 1940s and 1950s when the first investigations concerning the crystal structure of clathrate structures were carried out and structures I and II were identified. Similarly, it was discovered that mixed hydrates can be more stable and can have a melting point that is 10 to 15 °C higher. In the 1950s, the Dutch physicist Johannes Diderik van der Waals Jr, the son of Nobel Prize winner Johannes Diderik van der Waals, developed a thermodynamic model of methane hydrate together with J. C. Plateeuw.
Findings of natural methane hydrate
As early as the 1940s, there was speculation about natural methane hydrate deposits in the permafrost zone of Canada. At that time, the speculations could not yet be confirmed by findings. This changed in the early 1960s when Yuri Makogon discovered that methane hydrate occurs naturally in sediments. Russian drilling crews drilled a well in the upper reaches of the Messoyacha River in Siberia in the late 1960s, which encountered a deposit of methane hydrate for the first time in the upper part of a natural gas field. It was the first confirmation of the presence of natural methane hydrate. In the early 1970s, methane hydrate was detected in other Arctic areas, such as Alaska and Canada's Mackenzie Delta.
This transformed methane hydrate from a laboratory curiosity and operational hazard for natural gas pipelines into a potential energy source, and the discoveries triggered another wave of methane hydrate research. Of interest was the study of the geological and chemical parameters controlling the occurrence and stability of methane hydrate in nature, and the estimation of the volume of methane in the various methane hydrate deposits. At this time, initial investigations of the degradation behaviour began.
Deep Sea Drilling Project
The U.S. research vessel Glomar Challenger, a drilling platform for the study of methane hydrate, plate tectonics, and paleoceanography, extracted methane hydrate-bearing sediment from the deep sea during several expeditions in the 1970s and 1980s as part of the Deep Sea Drilling Project. The drilling program provided scientists with evidence of the existence of methane hydrate in a variety of geologic settings.
One mission objective of the Glomar Challenger was to investigate the nature of anomalous acoustic reflections detected at Blake Ridge, a deep area of the Atlantic Ocean that runs along the east coast of the United States. In the process, the geologists determined that methane hydrate deposits on the seafloor are detectable by reflection seismic techniques. When seismic travel times transition from a dense to a less dense medium, as occurs at the base of the methane hydrate stability zone, a so-called ground-simulating reflector characteristic of methane hydrate is created. This was confirmed by sediment cores that showed high methane concentrations. The deposits, which are located at a depth of more than 2500 meters below sea level at depths of about 700 to 750 meters below the seafloor, were estimated to contain 15 gigatons of carbon.
As part of the program, the scientists found methane hydrate in cores from the Central American Trench off Mexico and off Guatemala. They also detected methane hydrate deposits where no associated soil-simulating reflector was present. The results suggested that methane hydrate is found in continental margins around the world.
For the first time at that time, it was theorized that dissolving methane hydrate could be the trigger for submarine landslides, and that the decay of methane hydrate in the Earth's historical past could have led to a climate-influencing emission of methane into the atmosphere. Large-scale decay has been considered as an explanation for the Paleocene/Eocene temperature maximum.
At the end of the 1990s, initial tests began in Mallik on the Beaufort Sea to mine methane hydrate in the permafrost area there. Scientists from the USA, Europe, including Germany, Japan, India and China developed mining methods there.
Deepwater Horizon
In April 2010, an explosion occurred on the Deepwater Horizon, a drilling platform for oil exploration in the Gulf of Mexico. As a result, some 550 to 800 million litres of crude oil and about 147,000 tonnes of methane leaked into the sea, leading to the Gulf of Mexico oil spill, the worst environmental disaster of its kind in history. Methane hydrate may have been a contributing factor to the disaster. The well may have encountered methane hydrate in sediment that could have been broken down by a drop in pressure or heating. The methane could have possibly entered the well through a defect and contributed to high pressure in the well, which eventually led to the blowout that caused the rig to catch fire.
After the explosion, BP placed a dome weighing about 125 tons over the largest leak in the well. The dome was intended to collect the escaping crude oil and transport it via a pipe to a storage tank on the surface. However, under the prevailing temperatures and pressures, escaping methane formed methane hydrate with the seawater, which clogged the discharge pipe and thus impeded the outflow.
Production tests
A feasibility study tested the exchange of carbon dioxide for methane bound in methane hydrate at the Ignik-Sikumi No. 1 well in the Prudhoe Bay field in 2012. The released methane was produced by depressurizing the reservoir. Based on seismic surveys indicating methane hydrate deposits, Japan began test drilling in the northwest Pacific Ocean off Japan in late 1999. The drilling at a water depth of 945 meters confirmed methane hydrate deposits. Based on the results of the exploration, the Japanese government established a research program to study the mining of methane hydrate. In production trials in April 2017, methane was produced from methane hydrate for the first time in the open sea off Honshu Island using the pressure relief method. In the most successful well, a total of 222,500 cubic meters of methane was produced in 24 days.
A stratigraphic test well was drilled in Prudhoe Bay in 2018 and evidence of methane hydrate reservoirs was obtained. The well, named Hydrate-01, is intended as a monitoring well for long-term production testing. Among other things, the response of methane hydrate reservoirs to depressurization will be investigated.
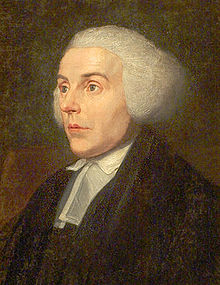

Joseph Priestley
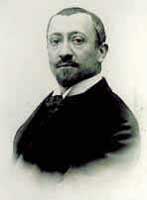

Paul Villard (around 1900)
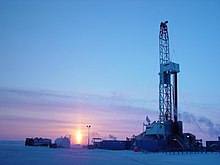

Mallik methane hydrate production exploration well, Canada
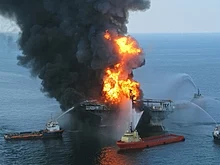

Fireboats fight the fire on the Deepwater Horizon oil rig

Research vessel Glomar Challenger
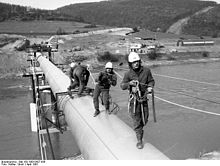

Urengoy-Uzhgorod natural gas pipeline in the Ukrainian oblast of Ivano-Frankivsk (1983)
Questions and Answers
Q: What is methane hydrate?
A: Methane hydrate is a combination of methane and frozen water, also known as methane ice or methane clathrate.
Q: How are the methane and water molecules arranged in methane hydrate?
A: The water molecules completely surround the methane molecules.
Q: Where is methane hydrate commonly found in the Earth's geosphere?
A: Methane hydrate is a common constituent of the shallow marine (ocean) geosphere.
Q: When was methane hydrate first discovered and where?
A: Methane hydrate was first discovered in 1971 in the Black Sea.
Q: What is the other name for methane hydrate?
A: Methane hydrate is also known as methane ice or methane clathrate.
Q: Which type of environment is methane hydrate most commonly found in?
A: Methane hydrate is most commonly found in shallow marine environments.
Q: What is methane?
A: Methane is a colorless, odorless gas and the main component of natural gas.