Kidney
The title of this article is ambiguous. For other meanings, see Kidney (disambiguation).
The kidney (Latin ren, usually only in the plural renes, derived adjective renalis; ancient Greek νεφρός nephrós) is a paired organ of the urinary system for the preparation of urine and regulation of the water and electrolyte balance in vertebrates. In the two kidneys, blood fractions below a certain size are filtered off, molecules important to the organism are largely reabsorbed, other substances are additionally secreted, and the aqueous solution is concentrated before being excreted. Diseases of the kidneys are mainly dealt with by nephrology as a branch of internal medicine and by urology.
Functions of the kidneys are:
- Elimination of end products of metabolism, called urinary substances, and toxins from the body through the formation of urine, which is finally excreted from the body through the urinary tract;
- Osmoregulation (balancing of the water balance);
- Volume regulation (long-term blood pressure control);
- Regulation and control of the composition of urine and control of electrolyte balance and acid-base balance.
Furthermore, the kidney is an important organ for the body's intermediate metabolism (it performs gluconeogenesis). The kidney also produces hormones, such as erythropoietin for blood formation, and is the site of degradation of peptide hormones. Conversely, many kidney functions are controlled by hormones; via renin, which is produced in the kidney itself, a sufficiently high blood pressure can be induced for its blood flow.
Each glomerulum (German: Nierenknäulechen) together with its Bowman's capsule forms a renal corpuscle (Corpusculum renale Malpighii). And each Malpighian renal corpuscle together with its associated tubule (German: Nierenkanälchen) forms a nephron (from ancient Greek νεφρός nephros, German 'kidney') as the smallest functional (morphological, physiological) subunit of the kidney of humans and other vertebrates.
The basic functional unit of the kidney is the nephron, which consists of renal corpuscles and renal tubules. Each human kidney has about one million nephrons, and thus just as many renal corpuscles, renal tubules and renal tubules. The renal tubules are sometimes described as urinary tubules because the primary urine is concentrated in them to form the secondary urine (or final urine); by analogy, the renal tubules are explained as filtering bodies.
The basic functioning of a nephron can be roughly divided into two processes:
In the first process, which takes place in the renal corpuscle, primary urine is squeezed out of the blood by cross-flow filtration. During this filtration, components above a certain size, including the blood cells and larger molecules, are retained. Thus, the ultrafiltrate contains only the low-molecular-weight components of the blood plasma, including those that are to be excreted. However, this primary urine also - and predominantly - contains numerous substances that are valuable for the body. Galenos was already thinking about renal filtration. William Bowman proved that glomeruli and tubules form a functional unit.
In a second process, which subsequently takes place in the renal tubules, valuable substances such as sugar, amino acids and electrolytes are reabsorbed back into the bloodstream in a controlled manner (reabsorption). Furthermore, a large part of the filtered water is also reabsorbed, which should not be lost to the body. These processes of reabsorption take place in various sections of the connecting, tubular tubule system - and additionally such active secretion of excretory substances into the urine. This interplay of reabsorption and secretion is referred to as tubular transport; in a sense, the tubules thus decide on the urinary duty of the individual electrolytes. The renal tubules thus concentrate the primary urine into the secondary urine (final urine), which collects in the renal pelvis, the beginning of the urinary tract.
From here, the urine is continuously passed through the ureter to the bladder. From the bladder, it is occasionally excreted via the urethra.
Every day, about 1800 liters of blood flow through the kidneys in an adult human (renal blood flow), which is about 300 times the blood volume of the body. From this, the two organs filter about 180 liters of primary urine daily (glomerular filtration), which is concentrated into less than two liters of final urine.
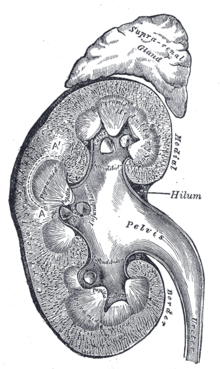

Cross section of the kidney (with adrenal gland)
Macroscopic anatomy
In humans
Location and neighborhood relations
In humans, the kidneys are located retroperitoneally (behind the peritoneum), on either side of the spine, which they do not project forward, below the diaphragm, in the lumbar fossa. The kidneys lie at about the level of the twelfth thoracic vertebra to the third lumbar vertebra, the right one (because of the large right lobe of the liver) about half a vertebral height lower than the left one. The upper kidney poles (see under shape) are about 7 cm apart, the lower ones about 11 cm apart. The longitudinal axes of both organs consequently point upward toward the center of the body. The lower kidney poles are 3 cm away from the iliac crest on the right side in males, 4 cm on the left side, and 2.5 cm and 3 cm away from the iliac crest in females, but can variably reach the iliac crest. The position of the kidneys is dependent on breathing. They move caudally during inspiration, as does the diaphragm. In the newborn, the kidney is always comparatively larger than other structures and therefore regularly overhangs the iliac crest.
The kidneys have - except directly to the adrenal glands - separated by the fat capsule contact surfaces to several organs of the abdominal cavity. The contact surfaces differ in the left and right kidneys: the left kidney is overlaid by the stomach, spleen, the splenic vessels (A. and V. splenica), the tail of the pancreas (cauda pancreatis) and the colon transversum. It forms part of the dorsal surface of the bursa omentalis with a triangular area in contact with the peritoneum. The right kidney is overlaid primarily by the liver, but also by the colon and duodenum (pars transversum duodeni). Because of the space taken up by the right lobe of the liver, which is located on the right side of the body (with the impressio renalis), the right kidney is located lower than the left. The crescent-shaped adrenal gland sits on top of both kidneys like a dome.
The subcostal nerve, iliohypogastric nerve and ilioinguinal nerve run across the back of the kidney in close proximity and can be affected by diseases. This can lead to sensations that are assigned to the innervation areas of these nerves, including pain in the lower abdomen.
Shape, color and size
The kidneys are bean-shaped and brown-red. They have a length of 10 to 12 cm, a width of 5 to 6.5 cm and a thickness of 3 to 5 cm (note: 12 cm × 6 cm × 3 cm). The mass of a kidney varies from 120 to 200 g. Usually, the left kidney is slightly larger and heavier. If one kidney is significantly reduced in size or missing, the other is usually enlarged. In humans, two so-called kidney poles point upward and downward, two surfaces point anteriorly and posteriorly (ventrally and dorsally), and two margins point medially and laterally. The outward facing margin is convex, the medial facing margin is concave and forms a recess in which the hilum renale, the entry and exit port of the conducting pathways, is located.
Renal hilus and conduction pathways
At the renal hilus, the renal vein, renal artery and ureter branch from ventral to dorsal, as do some lymphatic vessels and nerves. The hilus widens inside the kidney into the renal sinus, which is filled by the renal pelvis (urinary tract) and fatty tissue.
Each kidney is supplied with blood by usually one (very rarely several) renal artery arising directly from the aorta. The renal artery branches off from the aorta on both sides at the level of the superior mesenteric artery, points downward and divides already before the hilum into an anterior and posterior main trunk (ramus anterior et posterior), which are named after their position in relation to the renal pelvis and deliver the segmental arteries:
Four segmental arteries, the superior segmental artery, anterior segmental artery, inferior segmental artery, arise from the anterior main trunk anterior to the hilus. The posterior main trunk gives off an A. segmenti posterioris and supplies only one segment at the back of the kidney. The arteriae segmentorum are followed by the arteriae interlobares, then the arteriae arcuatae, then the arteriae interlobulares (also arteriae corticales radiatae), which finally give off the vasa afferentia for the renal corpuscles of the nephrons. For a more detailed description of the arterial supply, see the section on fine structure and the article Nephron.
The renal artery and each of its terminal branches are endarteries; no anastomoses are present, so that occlusion of one branch at a time leads to death of the renal tissue it supplies (necrosis, renal infarction).
The renal vein carries blood directly to the inferior vena cava. In the body, the aorta lies on the left and the inferior vena cava on the right, which is why the left renal vein is longer than the right. It lies in front of the aorta, below the outlet of the superior mesenteric artery (→ nutcracker syndrome) and receives the testicular or ovarica sinistra vein.
Urine released from the kidney into the renal pelvis is transported through the ureter to the urinary bladder.
Lymphatic capillary networks inside the kidney collect the lymph of the kidney and form few hilar lymphatic vessels at the hilus.
The sympathetic nerves of the kidney originate as postganglionic fibers from the coeliac plexus and run with the renal artery. They supply the pain-sensitive capsule in addition to the renal parenchyma. The parasympathetic nerves of the kidney originate as rami renales directly from the vagus nerve (X. cranial nerve).
Envelopes
The sheaths of the kidneys include the capsula fibrosa, capsula adiposa and fascia renalis (= Gerota's fascia):
Both kidneys are each encased in a thin, firm and smooth connective tissue organ capsule (capsula fibrosa). It contains very few elastic fibers and is hardly stretchable.
Together with the adrenal glands, a loose adipose tissue body of building fat follows, the capsula adiposa, which embeds and cushions the kidney. The capsula adiposa is more developed dorsally and laterally than abdominally and continues into the fat of the renal sinus inside the kidney. The fat body can be broken down in severe malnutrition.
All this envelops the renal fascia, a fascial sac that encloses the kidney, adrenal gland and fat body anteriorly, laterally and posteriorly, but is unclosed medially above and below. Behind the renal fascia sac lies the retrorenal fat (massa adiposa pararenalis), in which nerves of the lumbar plexus run.
Internal structure: rind and pith
The renal parenchyma, the actual organ mass of the kidney, is divided into the outer renal cortex (cortex renalis) and the renal medulla (medulla renalis), which is directed inward toward the hilum. The medulla has the shape of pyramids (10 to 12 medullary pyramids or renal pyramids) with their base pointing outward and their tip pointing inward toward the hilum. These tips, the papillae, extend freely into the cavity of the renal calices (calix renalis), which join together in variable form to form the renal pelvis (pelvis renalis), from which the ureter emerges. In this arrangement, urine flows from the papillae toward the ureter.
The renal cortex lies like a cap between the bases of the medullary pyramids and the organ capsule (subcapsular portion), but reaches the renal sinus between the pyramids in columnar sections (columnae renales, also called columnae renales Bertini or Bertin's columns after the French anatomist Exupère Joseph Bertin since 1744). The subcapsular portion of the cortex is traversed by clearly visible, fine lines, the medullary rays (radii medullares), which radiate from the medullary pyramids in the direction of the organ capsule and are part of the medulla. In the medulla itself, an outer medulla, consisting of an outer and an inner stripe, and an inner medulla located toward the renal pelvis can be distinguished by their slightly different color.
The structural division of the renal medulla into inner and outer zones as well as the division into inner stripes and outer stripes of the outer zone was first described by the anatomist Karl Peter (1870-1955) on the basis of investigations initiated by his teacher Philipp Stöhr in Würzburg and begun as early as 1904/05.
In other mammals
The principle position is also typical in the other mammals, here the kidneys are located behind the diaphragm (according to the horizontal body orientation). In many mammals, the right kidney lies somewhat further forward. In ruminants, the left kidney is displaced to the right, behind the right kidney, due to the formation of the pancreas (physiological migrating kidney).
In individual mammals, the kidney has a different structure. In its simplest form, the kidney consists of individual, cone-shaped renal lobes (lobi renales). This multi-lobed kidney is typical of marine mammals and bears. Each renal lobe consists of a cortical cap and a medullary pyramid that terminates in a renal papilla (papilla renalis, the pointed end of the cone).
In most mammals, these renal lobes (6 lobes in humans) fuse to varying degrees. The fusing cortical lobes form the renal cortex (cortex renis), and the pyramids form the renal medulla (medulla renis).
In cattle, only the middle parts of the individual kidney lobes fuse, creating furrows on the surface and preserving the kidney papillae as well. This type of construction is called multiblack furrowed kidney. This form also occurs in the meantime in the fetal development of the kidney in mammals, which are characterized by further fusion processes. The human newborn also still has a multiblack furrowed kidney.
In primates (including humans) and pigs, the cortical parts fuse completely after birth, so that the organ surface appears smooth. However, the individual papillae remain. This is referred to as a multi-black smooth kidney.
In most mammals, the individual renal papillae now also fuse to form a renal crest (crista renalis), so that the kidney is referred to as a one-black smooth kidney.
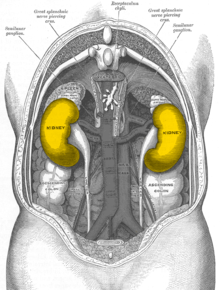

Position of the kidneys, seen from behind. The color is symbolic and does not correspond to reality.
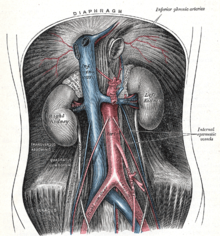

The aorta in the abdominal cavity with outlets (red)
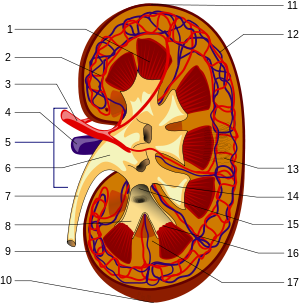

Schematic of the macroscopic structure of the kidney: 1. Renal medulla with medullary cones (pyramides renales) 2. Vas afferens3 . Renal artery (Arteria renalis) 4. Renal vein (Vena renalis) 5. Hilum renale6 . Renal pelvis (Pelvis renalis) 7. ureter (Ureter) 8. renal calices (Calices minores renales) 9. renal capsule (Capsula fibrosa renalis) 10. lower renal pole (Extremitas inferior) 11. upper renal pole (Extremitas superior) 12. vas efferens13 . Nephron14 . Renal sinus (sinus renalis) 15. Major renal calices (calices majores renales) 16. Medullary cone tips (papillae renales) 17. Column of Bertin (columna renalis)
Feinbau
The fine structure of the kidney is characterized by a highly differentiated tubular system and a specifically adapted blood supply. Based on embryonic development, the tubular system can be divided into two parts, the nephron and the collecting tube. Both form a functional unit. The last section of the nephron, i.e. the section near the collecting tube, is embryologically associated with the collecting tube.
Blood supply to the kidney
The kidneys are normally perfused by about 20% of cardiac output (i.e., about 1000 ml/min in adult humans). Approximately 20% of renal plasma flow is filtered into Bowman's space. Thus, renal perfusion leads to glomerular filtration (GFR). That is why GFR is largely proportional to cardiac output HZV. That is why the stage of renal failure is basically never smaller than the stage of cardiac failure.
Branches
The segmental arteries (see above) divide further. One interlobar artery supplies each of two adjacent medullary pyramids and corresponding cortical areas. It runs in the bark columns along the sides of the pyramids toward the bark, but branches at the base of the pyramid into arteriae arcuatae. These run in an arc at the medullary-cortical boundary and give off at right angles the arteriae corticales radiatae, which run radially upward through the bark, and also at nearly right angles in the direction of the medulla, the arteriae rectae.
First and second capillary bed
These eventually give rise to the vasa afferentia, each of which divides into a capillary cluster, the glomerulus (see below). From this first capillary area, the blood, which is still rich in oxygen, converges again in the vasa efferens. From there, the blood enters a second capillary bed, this time to supply the renal tissue. Here, one must distinguish two cases depending on the location of the glomerulus: From superficial glomeruli, located in the upper part of the cortex toward the organ capsule, the blood enters the peritubular capillary network of the cortex, which spins around the tubules located there. From juxtamedullary glomeruli, however, which lie lower toward the medullary-cortical boundary, arise the vessels supplying the medulla.
The supply of the Marks
These capillary vessels supplying the medulla are the vasa recta, which descend quite straight often to the tip of the papilla and ascend again in the opposite direction. There are numerous cross connections between descending and ascending limbs. The particular vascular architecture of the medulla is of great functional importance to the kidney's ability to concentrate urine. Using the countercurrent principle, the kidney generates a substantial osmotic gradient toward the tip of the papilla (see below), which would be washed out if the medulla were supplied with a normal capillary network. The price, however, is a very poor oxygen supply to the renal medulla, since oxygen from the oxygen-rich, descending limb of the vasa recta can diffuse directly into the ascending, oxygen-poor limb already at the top.
Venous system
Both capillary networks finally reach the venous system of the kidney, which - with the exception of the glomerula and its afferent and efferent arterioles - is analogous to the arterial system.
Nephron
→ Main article: Nephron
The kidney consists of numerous units, the nephrons, in which urine is formed. Each of the human kidneys contains 1 to 1.4 million nephrons. The nephron itself consists of a renal corpuscle (corpusculum renis) and a tubule apparatus.
The renal corpuscle contains the glomerulus (also called the glomerulum), a tangle of vessels through whose fenestrated capillary walls the primary urine is filtered. The primary urine passes from the renal corpuscle at the urinary pole (see figure) into the proximal tubule and into Henle's loop, where it is concentrated according to the countercurrent principle. This is followed by the distal tubule and a collecting tube (tubule renalis colligens).
·
Glomerulus in scanning electron microscope (SEM)
Image width approx. 115 µm
·
Glomerulus in SEM image width
approx. 22.8 µm
·
Glomerulus with broken capillary in SEM image width
approx. 11.5 µm
·
Interior view of the fenestrated endothelium (fenestrae) in the glomerulus of mouse kidneys in SEMImage width
approx. 1.1 µm.
.jpg)

Light microscopic sectional preparation of the renal cortex. * marks the urinary pole (see text) of a renal corpuscle.
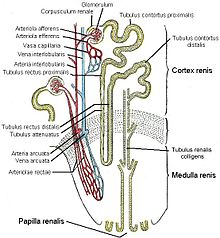

Fine structure of the kidney, schematic
Development
During embryonic development, three kidney generations develop in amniotes (umbilical animals): Pronephros, Mesonephros, and Metanephros. The pronotal kidney does not yet assume any function in the embryo. This task is first started by the primordial kidney and taken over by the after kidney. The tissue of the after-kidney eventually grows into the final kidney.
The after-kidney develops from two anlagen: the metanephrogenic blastem, the later urinary section, and the ureteric bud, the later urinary section that controls the amount of urine. The former gives rise to the renal parenchyma with the nephrons into which the branches from the aorta sprout. Persistence of the fetal blastemal tissue may lead to nephroblastomatosis.
The ureteral bud gives rise to the ureter, the renal pelvis with the renal calices, the collecting tubes, and the last segments of the nephron adjacent to the collecting tube.
The kidneys experience an ascension (ascensus) due to the growth in length of the embryo. In the process, they shift upward from the pelvic region. If the two lower kidney poles fuse, a single horseshoe kidney can develop. If a kidney fails to ascend, it remains in the pelvic region (pelvic kidney). If the kidney rises too high, it may lie in the thorax (intrathoracic kidney).
Initially, multiple mesonephric arteries supply the urinary kidney, most of which regress and usually only one renal artery remains. However, a second renal artery is relatively common. Accessory renal arteries are said to occur when an additional artery opens into the hilus, and aberrant arteries are said to occur when the vessel does not open at the hilus but independently, often at one pole. More than two renal arteries may occur, but are very rare.
Function
Kidney functions
The kidney is involved in the following body functions:
- Regulation of the water balance of the body.
- Long-term regulation of blood pressure.
- Excretion of urinary (e.g. uric acid, urea, creatinine) and toxic (e.g. drugs) substances.
- Regulation of the acid-base balance of the body. The pH value of the blood may only fluctuate within a narrow range, since larger changes in the direction of acidic or alkaline values lead to death.
- Regulation of the content of dissolved electrolytes in the blood (homeostasis): sodium, potassium, calcium, magnesium, phosphate, bicarbonate.
- Formation of various hormones: Renin (enzyme, short-term blood pressure regulation), erythropoietin (stimulation of blood formation), calcitriol (vitamin D, involved in calcium metabolism), kinins and prostaglandins.
- Significant involvement in the synthesis of glucose (dextrose) as gluconeogenesis, in addition to the liver.
Measurement of kidney performance
The function of the kidney can be estimated from the amount of urine, urine concentration and the concentration of urinary substances (creatinine, urea, uric acid, potassium) in the blood.
The exact performance of the kidneys is determined by renal clearance. There are various methods for this:
- Renal clearance is a measure of the elimination of a substance from the blood plasma and thus of the kidney's clarification function. If the clearance decreases, i.e. the performance of the kidney decreases, this is referred to as renal insufficiency.
- Inulin clearance measures the filtration capacity of the kidney. For this purpose, inulin is administered to the patient and it is measured how much of the administered substance is excreted again per time. Since inulin is filtered but not reabsorbed, the inulin clearance is identical to the glomerular filtration rate (GFR). For the healthy adolescent, the value is approximately 125 ml/min. A decrease in the value indicates a disturbance in renal function (renal insufficiency). With increasing age, the GFR physiologically decreases to 60-65 ml/min. This is important when dosing drugs that are excreted by the kidney, as older patients often require a reduction in dose due to lower GFR.
- Creatinine clearance is preferred to inulin clearance because of its simpler performance in the clinic. It measures the excretion of creatinine, which approximates that of inulin. Plasma creatinine levels, the value of which depends on muscle mass, vary little, which makes this measurement possible in the first place. Furthermore, it is advantageous that the infusion, which is required for the measurement of inulin clearance, is not necessary.
- With any reduction in cardiac output, the tubules compensatorily increase the reabsorption of water with all electrolytes dissolved in it to the point of oliguria or anuria. Therefore, in any heart failure and in any oligoanuria, the glomerular filtration rate cannot be validly determined by any method; the only exception is cystatin C. Cystatin C is constantly produced in the cells of the body and is freely filtered in the glomeruli. It is subsequently reabsorbed tubularly, but then completely destroyed while still in the tubules. It therefore does not return to the blood circulation. Therefore, it is a better filtration marker than creatinine or urea, especially in cases of mild renal impairment and increased muscle mass or acute renal failure. There are numerous GFR estimation formulas asking for cystatin C; the simplest is GFR = 80/Cys.
Autoregulation of renal blood flow
The driving force of the filtration process is the blood pressure prevailing in the glomerulus vessels of the first capillary bed. The (systemic) blood pressure of the body is normally subject to typical fluctuations during the course of a day, is lower during sleep, higher during physical exertion or stress, and can be permanently elevated in certain diseases (arterial hypertension). For filtration in the glomeruli, a sufficient pressure is necessary, favorably only slightly fluctuating. The kidney itself has the ability to regulate blood pressure in the glomerular capillary network even without nervous impulses and to keep the glomerular filtration rate so largely constant that even strong fluctuations in systemic blood pressure have little effect. This autoregulation of the kidney is called the Bayliss effect.
Autoregulation is mediated locally by pressure sensing and occurs through matched changes in vascular tension or vessel width in the afferent and efferent blood vessels of the renal corpuscle. When systemic blood pressure rises, the renal arteries are made narrower so that renal blood flow barely increases and pressure does not become excessive in the underlying afferent vessels of the renal corpuscle. If the filtration pressure is too low, the resistance in the outgoing (efferent) vessel from the glomerulus is increased and at the same time lowered in the incoming one. Thus, the effective filtration pressure can also be regulated independently of renal blood flow. On average, the glomerular capillary pressure is approximately 50 mmHg.
Normal blood pressure fluctuations have hardly any effect on renal perfusion. In this way, even fluctuations in systolic blood pressure between 80 and 180 mmHg have no effect on glomerular filtration performance. In a sense, the kidneys with their sensitive pressure sensors constantly monitor the systemic blood pressure and can intervene in a regulating manner in the event of an excessive drop (cf. Blood pressure regulation of the kidneys).
Tubuloglomerular feedback (TGF)
Tubuloglomerular feedback (TGF) refers to a mechanism by which the filtration of a single nephron in the kidney is regulated. TGF postulates an inverse behavior of glomerular filtration and tubular reabsorption and thus, to a certain extent, a proportionality between primary urine formation and urine production.
When the NaCl content in the distal tubule (midpiece) increases, there is a reduction in the glomerular filtration rate of the same nephron via a sensory function of the macula densa, a part of the juxtaglomerular apparatus. This is realized by mesangium-mediated vasoconstriction (vasoconstriction) of the arterioles (vasa afferentia) leading to the renal corpuscle.
Strictly speaking, this is a physiological regulatory mechanism that is intended to protect the solitary epithelium from hyperfiltration and is "mistakenly" activated in acute renal failure by the fact that NaCl reabsorption is severely impaired by tubular damage. This leads to an increased flow rate in the distal tubule and/or an increased NaCl supply in the region of the macula densa, which eventually leads to the triggering of the tubuloglomerular feedback.
Methods of examination of the kidney
- Physical examination
- Touch
- Knock
- Laboratory tests
- Urinalysis
- Test strips for nitrite, leukocytes, protein, blood, sugar, etc.
- Urine sediment
- Creatinine clearance
- Electrolytes
- The urine is also assessed by visual inspection and by odor
- Blood test
- Creatinine
- Potassium
- Urea
- Uric acid
- Cystatin C
- Stone examinations
- Imaging
- Ultrasound
- X-ray contrast imaging of the kidney = iv pyelogram
- CT of the kidney
- Magnetic resonance imaging of the kidney
- Angiography of the kidney
- Nuclear medicine procedures
- Static renal scintigraphy
- Renal excretory scintigraphy
- Renal perfusion scintigraphy
- Histological examinations
- Biopsy
Diseases of the kidney
Pathological changes in the renal tissue can affect the glomerula (glomerulonephritis) or the renal tubules (tubulointerstitial kidney disease) in acute kidney disease or chronic kidney failure. In the former, more autoimmune processes play a role, in the latter intoxications and infections (acutely mainly bacterial infections). In addition, both can be affected by autoimmune or metabolic systemic diseases. Genetic diseases mostly affect the function of the tubules. The various processes hardly differ clinically; a distinction is made between acute and chronic renal failure or acute and chronic glomerulonephritis. If left untreated, they lead to glomerulosclerosis and renal insufficiency requiring dialysis. There are also anomalies, renal tumors, renal stones.
Severe damage to the kidneys, on the other hand, results in disorders of blood pressure and hormone regulation of the organism. This leads to renal hypertension, renal vitamin D deficiency and secondary hyperparathyroidism, and in severe chronic renal insufficiency to uremic syndrome with organ damage and, among other things, itching. The damage can possibly be slowed down by a low-salt and low-protein diet and plenty of drinking, or dialysis therapy becomes necessary.
Systematics
- Malformations of the kidneys
- numerical anomalies: e.g. missing or extra kidney, see renal agenesis, renal hypoplasia
- Positional, fusion, and rotational anomalies: renal malrotation, wandering kidney, crossed renal dystopia, horseshoe kidney, malrotation of kidneys.
- Malformations of the renal vessels
- Malformations of the calyx system: e.g. calyx diverticulum or megacalicosis.
- Hereditary or genetically caused cystic kidney disease:
- Autosomal recessive polycystic kidney disease (ARPKD) → cystic kidney
- Autosomal dominant polycystic kidney disease (ADPKD) → Cystic kidney
- juvenile nephronophthisis
- medullary cystic disease
- congenital nephrosis syndrome
- Malformation syndromes such as Von Hippel-Lindau syndrome, tuberous cerebral sclerosis
- Non-hereditary cystic kidney disease:
- Simple renal cyst
- parapelvic renal cysts
- benign multilocular renal cyst
- multicystic kidney dysplasia
- Medullary sponge kidney
- Glomerulonephritis / glomerulopathy (autoimmune inflammation of the kidneys)
- acute (with nephritic syndrome)
- Rapidly Progressive Glomerulonephritis
- postinfectious glomerulonephritis
- chronic (with nephrotic syndrome)
- C1q nephropathy
- IgA nephritis
- focal segmental glomerulonephritis, see under nephrotic syndrome
- membranous glomerulonephritis
- membranoproliferative glomerulonephritis types I, II, and III, possibly due to Alport syndrome (defect of type IV collagen, associated with hematuria, progressive renal failure, and sensorineural hearing loss)
- Minimal Change Glomerulonephritis
- tubulointerstitial kidney disease
- acute
- bacterial (pyelonephritis, inflammation of the renal pelvis)
- viral (hantaviruses)
- parainfectious (Streptococcus' Epstein-Barr virus)
- Allergic/toxic
- chronic
- Analgesic anphropathy and other intoxications/hypersensitivity reactions.
- Sarcoidosis
- Systemic diseases with renal involvement
- Vasculitides (autoimmune vascular inflammations)
- Granulomatosis with polyangiitis
- Lupus erythematosus
- Purpura Schönlein-Henoch
- other vascular changes
- hypertensive nephropathy
- hemolytic uremic syndrome (HUS), thrombotic thrombocytopenic purpura (TTP)
- metabolic impairments
- diabetic nephropathy
- Amyloidosis
- multiple myeloma
- Gout
- hereditary kidney diseases
- tubular dysfunction
- Bartter syndrome (rare)
- Fanconi syndrome
- Renal tubular acidosis
- Phosphate diabetes
- congenital renal diabetes insipidus
- Diabetes renalis
- Cystinuria
- Balkan Nephritis
- Gitelman syndrome
- Fabry disease
- glomerular diseases
- familial focal segmental glomerulosclerosis
- Alport syndrome
- Thin basement membrane type glomerulopathy
- Kidney stones and nephrocalcinosis
- Kidney descent (colloquially "wandering kidney")
- Tumor
- malignant: renal cell carcinoma
- benign: e.g. angiomyolipoma, oncocytoma
- mechanical compression (Page kidney)
Syndromes
- Acute kidney failure
- chronic kidney failure
- cardiorenal syndrome
- hepatorenal syndrome
- nephrotic syndrome and nephritic syndrome
- renal hypertension
- renal anemia
- nephrogenic vitamin D deficiency (secondary hyperparathyroidism)
- renal osteodystrophy
- Uremia
- Polyuria' nocturia' polydipsia' oliguria' anuria
Effect of the loss of a kidney
After loss of one kidney, for example after a nephrectomy (for example after an accident, because of hypernephroma or for kidney transplantation), the remaining kidney can reach up to 80% of the filtration capacity of both kidneys. This hyperfiltration is achieved by hypertrophy of the glomeruli. This does not adversely affect the remaining kidney for decades.
Kidney as food
→ Main article: Kidney (food)
Especially pork, veal and lamb kidneys are used as food. They are mostly prepared in the form of ragouts.
Questions and Answers
Q: Where are the kidneys located in vertebrates?
A: The kidneys are located in the abdomen.
Q: What is the shape of the kidneys?
A: The kidneys are shaped like beans.
Q: What is the function of the kidneys?
A: The function of the kidneys is to regulate the amount of water and salts in the blood and to make urine for removal from the body.
Q: What is the meaning of the adjective "renal"?
A: The meaning of the adjective "renal" is related to the kidneys.
Q: What is renal failure?
A: Renal failure is when the kidneys are sick and do not work.
Q: What is the meaning of the prefix "nephro-" in medical terms?
A: The meaning of the prefix "nephro-" in medical terms is related to the kidneys.
Q: What is a nephrologist?
A: A nephrologist is a specialist who studies kidneys.