Gravity
The title of this article is ambiguous. For other meanings, see Gravity (disambiguation) and Gravity (disambiguation).
Gravity (from the Latin gravitas for "heaviness"), also called mass attraction or gravitational force, is one of the four basic forces of physics. It manifests itself in the mutual attraction of masses. It decreases with increasing distance of the masses, but has unlimited range. Unlike electrical or magnetic forces, it cannot be shielded.
On Earth, gravity causes all bodies to fall "down", i.e. towards the centre of the Earth, unless prevented from doing so by other forces. In the solar system, gravity determines the orbits of planets, moons, satellites and comets, and in the cosmos, it determines the formation of stars and galaxies, as well as its evolution on a large scale.
Gravity is often equated with gravity. However, the force on a body (the weight of the body) determined by the locally prevailing gravitational field includes not only the gravitational force, but also the inertial effects acting on the body (in particular due to the rotation of the reference frame).
Within the framework of classical physics, gravity is described by Newton's law of gravitation, i.e. as a long-range force acting instantaneously, i.e. directly and without loss of time through empty space. A fundamentally different understanding of gravitation results from the general theory of relativity according to Albert Einstein. Here, gravity does not act in the form of a force on the bodies, but corresponds to a curvature of the four-dimensional space-time, whereby the paths of the bodies, on which no further forces act, correspond to a shortest line in the curved space, i.e. a geodesic.
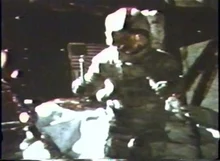

Play media file David Randolph Scott, commander of the Apollo 15 lunar mission (1971), uses a feather and a hammer dropped in a vacuum on the moon to demonstrate Galileo's law of falling, that all bodies fall at the same rate regardless of their mass.
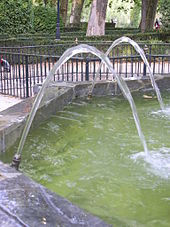

A jet of water from a fountain directed upwards at an angle deforms into a parabola on earth under the influence of gravity.
.jpg)

Two spiral galaxies deforming under the influence of each other's gravity
Historical overview
Ancient
In antiquity, the Greek philosopher Aristotle, in the context of his cosmology, described gravity as that property of the sublunary elements (earth, water, air, fire) which causes all bodies consisting of these elements to strive towards the centre of the world. This idea was for a long time the main physical argument for the geocentric world view.
Orient
Ancient Indian authors attributed free fall to a force proportional to the mass of an object and acting in the direction of the center of the earth. In the 9th century, the Persian astronomer Muhammad ibn Musa explained the movements of celestial bodies by a force of attraction. Al-Biruni translated the works of Indian authors into Arabic and Persian in the 11th century. His contemporary Alhazen formulated a theory of gravitational attraction. In the 12th century, the Persian Al-Khazini suggested that the strength of the Earth's gravitational pull depended on the distance from the center of the Earth and distinguished between mass, weight, and force.
Late Scholastic
An important critic of peripatetic (Aristotelian) physics and forerunner of the Copernican worldview is the late Scholastic Nicholas of Oresme. In the 14th century he considered the rotation of the earth to be probable and described the possibility of many worlds as well as many gravitational centres - in contrast to an earth at rest, lying at the centre of the universe and attracting everything heavy.
Copernicus
In 1543, Nicolaus Copernicus assumed in De revolutionibus orbium coelestium that, apart from the Earth, all other celestial bodies also exert gravitation:
"... I am at least of the opinion that gravity is nothing other than a natural striving implanted in the parts by the divine providence of the Master of the World, by virtue of which they form their unity and wholeness by joining together in the form of a sphere. And it is to be assumed that this tendency is also inherent in the sun, the moon and the other planets ..."
Kepler
Johannes Kepler published the following axioms in his Astronomia nova in 1609:
- Every corporeal substance, in so far as it is corporeal, is naturally inclined to rest in any place where it is alone, outside the sphere of force of a kindred body.
- Gravity consists in the mutual physical striving between related bodies for union or connection (of this order is also the magnetic force), so that the earth attracts the stone much more; than the stone strives after the earth.
- The heavy is [...] not driven towards the centre of the world as such, but as the centre of a related round body ...
- If the earth were not round, the gravity would not be driven everywhere in a straight line towards the centre of the earth, but from different sides towards different points.
- If one were to move two stones to any place in the world, close together outside the range of force of a third related body, then those stones would unite in a manner similar to two magnetic bodies at an intermediate location, one approaching the other by a distance proportional to the mass of the other.
- The range of the moon's gravitational pull extends to the earth.
17th century
Also in the early 17th century, Galileo Galilei described the free fall of a body as a uniformly accelerated motion that is independent of its mass or other properties.
In his 1636 work "Traité de mécanique des poids soutenus par des puissances sur des plans inclinés à l'horizontale" Gilles Personne de Roberval developed the idea of a gravitational force, years before corresponding publications by Robert Hooke and Isaac Newton. René Descartes explained gravity as a consequence of his theory of vortices. In 1644 he published the Principia Philosophiae, which had great influence - also on the criticism by Isaac Newton - because comets obviously could not be explained by Descartes' model. That comets penetrate or cross the spheres or orbits of the planets had been the prevailing opinion since Tycho Brahe and the comet of 1577.
The English scholar Robert Hooke explained the effect of gravity with "gravitational funnels" around 1670. He explained that gravity was a property of all massed bodies and was greater the closer two bodies were to each other. The theory that gravity is inversely proportional to the square of the distance from the center of mass first appeared in 1680 in a letter from Hooke to his compatriot Newton.
Newton
Isaac Newton was the first to describe gravity using a mathematical formula in his Principia (1687). This law of gravitation, which he formulated, is one of the basic equations of classical mechanics, the first physical theory that could also be applied to astronomy. According to it, gravity is a force between two bodies that accelerates them towards their common centre of gravity, its strength decreasing in proportion to the square of the distance between the bodies. Newton's theory, completed around 1800 by Pierre-Simon Laplace, provides a fundamental understanding of the dynamics of the solar system with the possibility of accurate predictions of the motion of planets, moons, and comets. It confirms Kepler's laws of planetary motion for individual planets, but in addition allows the perturbing influence of the other planets and moons to be determined. For a long time, the values calculated according to it were in perfect agreement with the corresponding astronomical and terrestrial observations and experiments. The first discrepancy that could not be explained in this way was discovered in the middle of the 19th century at the perihelion rotation of the orbit of Mercury.
Alternative theories in the 18th and 19th centuries
To explain gravity in terms of a process event, a number of mechanical, kinetic explanations continued to be proposed until the development of general relativity in the early 20th century (see Mechanical explanations of gravity). One of the best known is the theory of Le Sage gravity developed by Fatio and Le Sage. This argues that the gravitational attraction of two bodies is due to the shielding of the pressure acting from the direction of the other. Related to this are the theories of an ether as a mediator of interactions (rather than a long-range effect) such as the electromagnetic interaction. One of the last of these theories was Lorentz's aether theory, developed around 1900, which was eventually superseded by the novel approach of Einstein's theory of relativity.
Einstein
In the general theory of relativity (ART) established by Albert Einstein in 1916, gravity is attributed to a geometric property of spacetime. He assumes that spacetime is curved by the presence of mass and any form of energy. This makes it possible to interpret gravity in a fundamentally different way than the other forces, namely as an inertial force. According to the principle of equivalence, the effect of gravitation cannot be distinguished from the effect of acceleration of the reference frame; in particular, in a freely falling reference frame, the effects of gravitation and acceleration exactly cancel each other out. Gravitation is said to be "transformed away" by the transition to the new coordinates. However, this is only true for one location at a time (locally), because each real gravitational field causes different accelerations for neighboring locations, which cannot be "transformed away" at the same time. In general relativity, each point in space is assigned its own local inertial frame, where there is no gravity and where special relativity with its four-dimensional, flat spacetime applies. Analogous to the fact that, according to Galileo, force-free motions are rectilinear and uniform, in general relativity bodies without non-gravitational forces move on geodesics in a "curved" space with Riemannian geometry. The Einstein field equations are used to determine the curvature of spacetime prevailing at a point. They were formulated in such a way that in the limiting case of weak gravity the results calculated according to them agree with those calculated according to Newton's equation. General relativity thus treats gravity as an inertial force and places it on a par with centrifugal force, Coriolis force, or the force felt in a vehicle when starting or braking.
Within the solar system, where weak fields or a low curvature of space-time prevail, only small deviations from the predictions of Newton's law of gravitation result. The first successful example of the application of general relativity was the explanation of the small deviation between the observed perihelion rotation of the orbit of Mercury and the value predicted according to Newton's theory due to orbital perturbations by the other planets.
In the case of strong curvature, as caused by the concentration of a large mass in a small space, completely new phenomena such as black holes are predicted.
In Newtonian mechanics, mass alone is considered to be the source as well as the point of application of gravity. Starting from the originally imprecise concept of a given quantity of matter, mass got its first precise physical definition here. In general relativity, gravity is an expression of the curvature of space-time, which in turn is influenced not only by the presence of matter, but also by energy in every form, including gravitational energy itself, and moreover by mass and energy flows. All predictions of general relativity accessible to observation have been confirmed by measurements.
In Newtonian gravitation, one still assumed an instantaneous or instantaneous propagation of the gravitational effect, i.e. that the effect is instantaneous even over large distances. Within the Einsteinian view, however, it is true that no effect, including the gravitational effect, propagates faster than the speed of light. By a fast change of position of masses, as for example at fast circling double-stars or at collapse of a star, rather gravitational waves are produced, which propagate with speed of light.
Quantum Gravity
Not experimentally accessible are extremely high concentrations of mass or energy in a very small space, for the description of which quantum effects must be taken into account in addition to gravity. Attempts at a quantum field theory of gravity exist in rudimentary form. However, there is a lack of predictions that are both calculable and observable. The basic problem is that at such concentrations black holes are formed quickly, in whose interior quantum effects take place that elude observation.
Modified Newtonian Dynamics (MOND)
The phenomenon of "dark matter" stands for the difference between the observed masses and the masses expected according to the models of Kepler, Newton and Einstein in the rotational behaviour of galaxies and galaxy clusters. Instead of additional, unseen mass, Mordehai Milgrom proposed in 1983 that a change in Newton's laws of motion could be the cause of the observed rotation curves. According to the "MOND hypothesis", the change has a relevant influence on the motions only at very small accelerations, as they occur on the astronomical scale.
Proponents of "Modified Newtonian Dynamics" argue that Newton's 1686 theory of gravity has already undergone three modifications. At very small distances, physicists use only quantum mechanics; at very large velocities, Einstein's special theory of relativity; and near very large masses, his general theory of relativity.
.webm.jpg)

Play media file Video: Gravity as the curvature of space
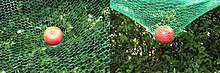

"Newton's apple bends spacetime," vividly. William Stukeley reports that Newton came up with gravity by a falling apple, see Memoirs of Sir Isaac Newton's life
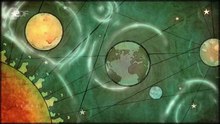

Play media file Animation: Newton's idea of gravity
Gravitation in classical mechanics
→ Main article: Gravitational field and Newton's law of gravity
In classical mechanics, gravitation or general mass attraction is a property of all matter that depends only on its mass, not on its nature or motion. Gravity is expressed in the gravitational force or gravitational field, which is generated by every mass, has an attractive effect on every other mass, and has infinite speed of propagation and range. Newton's law of gravitation gives the instantaneous force with which two point-like imaginary bodies with the masses
and
at a distance attract each other
(
is the universal gravitational constant):
This force is the same for both bodies, but directed in opposite directions. If no other forces act, each of the two bodies experiences an acceleration towards the other. This instantaneous acceleration can be
calculated using Newton's second law For example, it results for the body 1:
The instantaneous acceleration of body 1 therefore does not depend on its mass , but on the mass
the other body. Thus, at a certain distance, body 2 imparts the same acceleration to any other body, regardless of its mass. Conversely, the same applies to the acceleration that body 1 imparts to any other body at a distance :
The accelerations are therefore indirectly proportional to the accelerated masses: . Taking the Earth for body 2 and any everyday object for body 1 means that the Earth experiences only an immeasurably small acceleration due to its much larger mass from body 1. It can therefore be assumed to be at rest. However, body 1 experiences an acceleration from it that depends on the distance from the center of the Earth, but not on the mass
. This explains the fact, first stated by Galileo Galilei, that in empty space (i.e. unhindered by other forces or resistances) all bodies experience the same acceleration due to gravity regardless of their mass. The equality of gravitational acceleration is also known as the principle of equivalence of inertial and heavy mass (in its weak formulation).
If the masses of the two bodies are not as different from each other as in the preceding example, then both bodies perform accelerated motions, whereby the total centre of gravity between the two masses can be chosen as the stationary reference point (see centre of gravity theorem). If both bodies start from rest, they tumble towards each other in a straight line until they meet. (In the abstraction as point masses this would happen at the total centre of gravity).
However, if they each have an initial velocity in the center-of-mass system, they perform motions whose trajectories lie in a common plane; this is required by the law of conservation of angular momentum. The shape of these trajectories depends on the velocities of the two bodies (see two-body problem). One possible solution is elliptical orbits, where the center of gravity forms a focal point of each of the two ellipses. An example of this is the Earth-Moon system, where the mass of the Earth is so great that the common center of gravity even lies inside the Earth.
Systems consisting of three or more bodies that attract each other often behave chaotically and are not computable by analytical methods (see three-body problem). However, there are helpful approximations. In the solar system, for example, the mass of the planets is very small compared to the mass of the sun. If one assumes that the Sun is therefore not influenced by the planets and that the planets do not interact with each other, then calculations using Newton's law of gravity yield Kepler's orbital ellipses of the planets.
The classical description of gravity is therefore sufficiently accurate for many applications. However, deviations occur in connection with very precise measurements, e.g. the perihelion rotation of Mercury, and the classical description fails completely under extreme conditions, which exist, for example, in the case of black holes.
The weight of a body on the earth's surface is largely determined by gravity. In addition, inertial forces contribute to the weight force; for example, the centrifugal force resulting from the Earth's rotation counteracts gravity somewhat. Gravity and inertial forces together form the gravitational field.
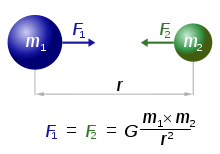

Newton's law of gravitation
Questions and Answers
Q: What is gravity?
A: Gravity, or gravitation, is one of the fundamental forces of the universe. It is an attraction, or pull, between any two objects with mass.
Q: How does gravity affect everyday life?
A: Gravity affects everyday life in that it causes objects to fall to the ground due to its force of attraction between two objects with mass.
Q: What are Newton's laws regarding gravity?
A: Newton's laws state that gravity keeps the Solar System and most major astronomical objects together.
Q: What is Einstein's theory of general relativity?
A: Einstein's theory of general relativity states that gravity plays a role in the universe by influencing how space and time interact with each other.
Q: Is there any evidence for what causes gravity?
A: Some physicists think that gravity may be caused by gravitons, but this has yet to be confirmed.
Q: How does gravity influence space and time?
A: According to Einstein's theory of general relativity, gravity influences how space and time interact with each other in the universe.