First law of thermodynamics
Thermodynamics (from ancient Greek θερμός thermós, German 'warm', as well as ancient Greek δύναμις dýnamis, German 'force') or heat theory is a scientific and engineering discipline. It originated in the study of steam engines and pursued the question of how to convert heat into mechanical work. To do this, it describes systems of sufficiently many particles and their state transitions using macroscopic state variables that are statistical functions of the detailed many-particle states. As an engineering science, it has significance for the various possibilities of energy conversion, and in process engineering it describes the properties and behaviour of substances involved in processes. The French physicist Sadi Carnot, who wrote his seminal work in 1824, is considered the founder.
The main theorems of thermodynamics are of great importance, occupying a similar position to Newton's axioms in classical mechanics or Maxwell's equations in electrodynamics. The first law states that the total energy in a closed system is constant and is valid as conservation of energy in all of physics. The second law expresses the direction in which energy transformations are possible. For example, it is possible to convert mechanical, electrical or chemical energy completely into heat energy (thermal energy). Thermal energy, on the other hand, can only be partially converted into these energies, and only with a great deal of technical effort.
There are two different approaches in thermodynamics, which differ in whether substances are viewed as a continuum that can be divided arbitrarily, or whether they are viewed as a collection of particles such as atoms or molecules:
- The older approach considers substances as a continuum and is called classical, phenomenological or engineering thermodynamics (also engineering thermodynamics) and uses terms such as heat, pressure, volume and temperature. It is part of classical physics and many engineering sciences. If the systems under consideration consist of at least
particles, which is always the case for engineering systems, this is a very good approximation.
- Statistical thermodynamics, on the other hand, starts from individual particles and describes them using statistical methods and the kinetic theory of gases because of their large number. It is therefore part of statistical physics and explains, for example, how the pressure of a gas on the container arises from collisions of the individual molecules of the gas or how the temperature is related to the kinetic energy of the particles. This approach thus serves as an explanation for various phenomena and as a theoretical foundation for the main theorems, but it offers no advantages for analysis or calculation in engineering, so it is not pursued there.
On the one hand, thermodynamics deals with various processes when heat is involved in them, without going into the specifics of the substances involved. Of particular importance are circular processes, which occur frequently in technology. On the other hand, it makes statements about substances such as the different states of aggregation and their change (melting, boiling, evaporation ...) or chemical reactions, which depend very much on the respective substances.
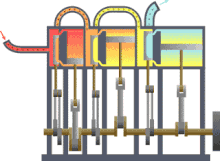

Typical thermodynamic process using the example of the principle mode of operation of an engine driven by steam (red = very hot, yellow = less hot, blue = final temperature of the medium)
Meaning
Within the natural sciences, thermodynamics is of great importance, since energy is involved in all processes occurring in nature. This also includes living beings. It also provides a deeper insight into the properties of matter, which on the one hand is helpful for understanding physical properties or changes in aggregate states, and on the other hand is important for understanding which chemical reactions can and cannot take place. Within physics, it is also emphasized that thermodynamics can connect different independently developed fields such as classical mechanics or quantum mechanics, which becomes possible in particular via the universal concept of energy.
In engineering, thermodynamics is important for the design, calculation and analysis of numerous machines or plants. These include the various heat engines (steam engine, gas or steam turbine, diesel engine), the working machines (pumps, compressors, ...), air conditioning and refrigeration, heat and mass transfer, industrial furnaces, supply and disposal technology or power engineering (power plants).
Important terms in thermodynamics
Thermodynamics relates the process variables heat and work at the system boundary to the state variables, which describe the state of the system.
On the basis of four fundamental laws as well as material-specific, empirical equations of state between the state variables (see e.g. gas law), thermodynamics allows statements to be made about which changes are possible in a system (for example, which chemical reactions or phase transitions can take place, but not how) and which values of the intensive state variables are required for this by establishing equilibrium conditions. It is used to calculate heat energy released, changes in pressure, temperature or volume, and is therefore of great importance for the understanding and planning of processes in chemical plants, in heat engines and in heating and air conditioning technology.
In order to describe systems and properties briefly and precisely, certain terms and agreements are repeatedly used in thermodynamics:
- Thermodynamics is primarily concerned with the entropy and thermal energy (heat energy) contained in any system. In an isolated system, the temperature is a measure of the contained thermal energy.
- Energy is understood in thermodynamics as the sum of two parts, namely exergy and anergy, whereby each part can be 0. The exergy can be converted into other forms of energy (technical work), i.e. perform work or be converted into anergy. Technical work is always pure exergy and thus entropy-free. Anergy can no longer be converted into other forms of energy.
- The environment or ambient state serves as a reference for the ability of a system to do work. Systems that are not in thermodynamic equilibrium with the environment still have exergy, while the energy of the environment is pure anergy.
- A state variable describes a property of the system. Extensive state variables such as internal energy U, entropy S, volume V and particle number N change when the system is divided. In contrast, intensive state variables such as temperature T, pressure p, concentration n and chemical potential μ remain the same.
- A process changes a system from one state to another. A process variable is, for example, a heat flow supplied or a power dissipated.
- If a state variable remains the same during a change of state (process), it is denoted by the prefix iso. Commonly used are isochoric (preserves the volume, as in the equal-space process), isobaric (preserves the pressure, as in the equal-pressure process), isothermal (preserves the temperature), isenthalpic (preserves the enthalpy) or isentropic (preserves the entropy, therefore reversible); an isentropic change of state occurs adiabatically and frictionlessly. Isentropic is not to be confused with isotropic!
- Closed or isolated systems exchange neither substances nor energy with their environment, closed systems can at least exchange energy, and both substance and energy flows pass through open systems.
- Adiabatic processes and containers are heat dense but can exchange substances and work. Work-dense or rigid systems do not exchange work with the environment (except shear work in steady-state flow processes) and diathermic systems only transfer heat to the outside.
- System boundaries or balance envelopes are virtual boundaries for analysing a system (material flow analysis). In a balance equation, the change of a state variable within the system boundary can be considered
- A reversible process can be reversed at any time without causing changes to the environment. Reversible processes form the theoretical boundary between possible/real and natural law processes. Reversible processes are isentropic and run both adiabatically and frictionless.
- An ideal gas is a simplified model for highly dilute gases in which the individual particles exert practically no cohesive effect on each other and in which the intrinsic volume of the gas atoms or molecules is negligible.
- If a real gas has the inversion temperature, it maintains its temperature during a throttling process. Below the inversion temperature it cools down due to the throttling, above it it heats up. Ideal gases maintain their temperature during throttling.
- Circular processes run cyclically and always return their working materials to the initial state. Left-running cycles consume work (refrigeration machines, heat pumps), right-running cycles supply work (heat engines).
- The Carnot process is an ideal circular process. Since heat can never be completely converted into technical work (exergy), the efficiency of the Carnot process indicates the maximum proportion of work that can be obtained from heat.
- Only at the triple point can solid, liquid and gaseous phases of a substance exist simultaneously. Since the triple point has a fixed pressure and temperature, triple points are suitable as reference points to calibrate a temperature scale. For the Kelvin scale, the triple point of water (0.01 °C) is used.
- At the critical point, the molecules of a substance are as densely packed in the gas as they are in the liquid. The critical point is determined by temperature, pressure and density. Above its critical temperature, a gas can no longer be liquefied by pressure.
- A fluid can be a gas or a liquid. In particular, when considering the transformation (phase transition) of a substance between liquid and gaseous, the term fluid simplifies the description, since it is not fixed to a phase.
In thermodynamic formulas, certain letters are repeatedly used for certain quantities. Capital letters in formulas denote an absolute quantity, for example V as a volume [m³]. Lower case letters denote specific quantities, for example v as a volumetric flow (volume related to a mass, [m³/kg]), a mass flow [kg/s] or a quantity of substance [m³/mol].
- The thermodynamic or absolute temperature T is measured in Kelvin (until 1954: degrees Kelvin). 0 Kelvin (-273.15 °C) cannot be reached by thermodynamic means (3rd law).
- The internal energy U is the energy stored as heat in the system, the enthalpy H additionally contains the energy stored in pressure × volume.
- The entropy S is a measure of disorder and at the same time a measure of the probability of a state. Entropy can never decrease in a closed system. Systems always strive of their own accord towards the state of the highest possible entropy (2nd law).
- Volume change work w = ∫ p-dv is performed when changing the volume against the prevailing pressures. These are, on the one hand, the pressure of the compressed fluid and the pressure of the environment.
- The amount of substance N is a measure for the number of particles and is measured in the unit mole.
- The heat capacity refers to the ability of a substance to absorb heat energy. The greater the heat capacity, the smaller the temperature change when heat is absorbed or released.
Questions and Answers
Q: What is the first law of thermodynamics?
A: The first law of thermodynamics is that energy can neither be created nor destroyed; it can change only from one form to another.
Q: What is the principle of conservation of energy?
A: The principle of conservation of energy means that anything that uses energy is changing the energy from one kind of energy to another.
Q: Can perpetual motion machines ever exist?
A: No, perpetual motion machines could never exist because it would break a fundamental law of physics, which states that energy can neither be created nor destroyed.
Q: What are examples of forms of energy in classical mechanics?
A: Examples of forms of energy in classical mechanics include heat, light, kinetic (movement) or potential energy.
Q: How many types of energy are there in modern physics?
A: In modern physics, it is considered that there are only two types of energy - mass and kinetic energy, although this may not be helpful to those not familiar with more complex physics.
Q: Is the total energy of the universe constant?
A: Yes, the total energy of the universe (or any Closed system) is a constant. However, energy can be transferred from one part of the universe to another.
Q: What is the most common wording of the first law of thermodynamics used by scientists?
A: The most common wording of the first law of thermodynamics used by scientists is that energy cannot be created or destroyed; it can only be transferred or converted from one form to another.