Ferromagnetism
Ferromagnetism (from Latin ferrum 'iron') is the best known type of magnetism of solids. It is explained by the fact that the magnetic moments (elementary magnet) of the atoms of the material tend to align in parallel. Ferromagnets either generate a permanent magnetic field themselves or are strongly attracted to one pole of an external magnetic field. Ferromagnetic materials are usually solids. Known applications include permanent magnets, electric motors, transformers, and the various forms of magnetic data storage (magnetic tape, floppy disk, hard disk drive).
As pure metals, iron, cobalt and nickel are ferromagnetic at room temperature (approx. 20 °C). At lower temperatures, some lanthanides are also ferromagnetic, e.g. gadolinium at up to 19.3 °C.
Ferromagnetic materials magnetize in an external magnetic field in such a way that the magnetic flux density inside them increases compared to outside space, and are thus attracted towards higher field strengths ("into the magnetic field"). The factor of flux density increase compared to empty space is described by the magnetic permeability μ (or the magnetic susceptibility μ
) of the material is described; for ferromagnets, μ
. Other types of stable magnetic order of elementary magnets are antiferromagnetism and ferrimagnetism.
An increase of the flux density in the material also occurs with paramagnetic materials. However, they do not produce a stable long-range order of the magnetic moments. Therefore, the effect is usually weaker.
Ferromagnets show a tendency to maintain their magnetic order even against external influences. This leads, among other things, to the fact that they retain the magnetic order generated inside and thus the external magnetic field generated by them, even if they are no longer exposed to a magnetic field. This tendency is called remanence of ferromagnetism. It is caused by effects in two different orders of magnitude:
- microscopic: the rectified magnetic order of the elementary magnets (e.g. of the electron spins, see below) on an atomic scale
- macroscopic: the arrangement of the Weiss districts (so-called "domains") in the order of micrometres to nanometres
Many considerations in theoretical solid state physics are limited to the microscopic aspect and already call the rectification of elementary magnets ferromagnetism. On the other hand, the Weiss domains also occur in other magnetic orders.
Schematic progression of magnetic induction B from ferromagnetic (), paramagnetic ( ),
and diamagnetic materials (
) to vacuum (
).
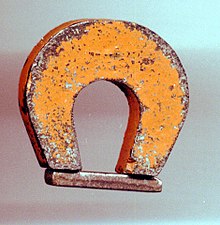

By ferromagnetism the lower iron piece is attracted by a horseshoe magnet.
Introduction
A material is classified or declared as a ferromagnetic material when, below the Curie temperature, the magnetic moments of the atoms align in parallel in it. This effect is due to the fact that in these materials there is an interaction between the atoms which causes the total energy of the material to be reduced by ordering compared to a disordered configuration.
This tendency of the elementary magnets to align themselves in parallel leads to a spontaneous magnetization of larger areas, the white districts, in which the elementary magnets are mostly aligned in parallel. This distinguishes ferromagnets from paramagnets, where the magnetic moments are normally disordered.
In the absence of external influences, the directions of the magnetic fields of adjacent white districts are anticorrelated. In the Bloch and Neel walls between the districts, the elementary magnets are aligned in such a way that a transition occurs between the two directions of magnetization. In this state, a body made of a ferromagnetic material does not generate an external magnetic field, since the fields of the different Weiss domains compensate each other.
When the material is subjected to an external magnetic field, the Weiss districts, which are magnetized in the opposite direction to the external magnetic field, shrink and eventually fold over. This creates a macroscopic magnetization whose field overlaps with the external one in such a way that the field lines appear to be drawn laterally into the material. In an inhomogeneous field, the material thus magnetized is attracted to sites of greater field strength, be they magnetic north or south poles. Paramagnets behave similarly, but the alignment of the magnetic moments occurs solely due to the external field and not additionally due to the parallelizing influence of the neighboring moments. Therefore, the effect is much weaker.
Ferromagnetic materials are classified according to the behaviour they exhibit when removed from a magnetic field. Generally, a residual magnetism then remains, the so-called remanence.
- In soft magnetic materials the remanence is low, i.e. most of the magnetization is lost immediately when the object is removed from the external magnetic field, especially after alternating fields have been applied.
- Hard magnetic materials are harder to magnetize but retain a greater permanent magnetization. Such materials, e.g. hardened steel, can be magnetized into permanent magnets or exist as permanent magnets from the outset, i.e. permanently assume a clearly recognizable (macroscopic) magnetization.
The remanence magnetization can be eliminated by applying a magnetic counterfield, which occurs when the coercive field strength is reached. In the case of hard magnetic materials, the level of the necessary counter-field is greater than in the case of soft magnetic materials. In the case of permanent magnets, both a high remanence and a high coercivity are desirable.
Ferromagnetism must be distinguished from ferrimagnetism (e.g. in ferrites), which has macroscopically similar properties but is microscopically related to antiferromagnetism. In this case, as in antiferromagnetism, the elementary magnets are alternately directed in opposite directions, but with different strengths in the two directions, which is why - unlike in antiferromagnetism - a magnetization remains for each pair.
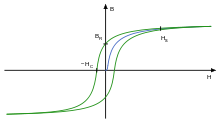

Typical hysteresis curve of ferromagnetic systems : The new curve (first-time magnetization!) is shown in blue, the curves with repeated alternating magnetization are shown in green. The remanence magnetization and the coercivity (the axis values!) are marked.
Substances with ferromagnetic properties
Substance |
|
Co | 1395 |
Fe | 1033 |
Ni | 627 |
CrO2 | 390 |
Gd | 289 |
Tb | 219 |
Dy | 85 |
EuO | 70 |
Ho | 20 |
He | 19 |
Among the elements or metals in pure form, iron, nickel and cobalt exhibit ferromagnetic properties at room temperature. In 2018, ruthenium was identified as the fourth element with ferromagnetic properties at room temperature (in the metastable body-centered tetragonal phase). At lower temperatures (see table), the lanthanides gadolinium, terbium, dysprosium, holmium and erbium also become ferromagnetic.
In practice, ferromagnetic alloys such as AlNiCo, SmCo, Nd2Fe14B, Ni80Fe20 ("permalloy"), or NiFeCo alloys ("mumetal") are often used. It is noteworthy that under certain circumstances also some compounds of generally non-ferromagnetic elements exhibit ferromagnetic behaviour, for example chromium dioxide, manganese arsenide, europium(II) oxide or the superfluid A-1 phase of He-3, furthermore the so-called Heusler alloys.
It is also worth noting that the best known ferromagnetic material, iron, is not ferromagnetic as the main component of an austenitic alloy. Austenitic microstructures are a component of many stainless steels and some grades of stainless steel. (Iron crystallizes in the body-centered cubic lattice at room temperature. Austenitic alloys, on the other hand, are predominantly face-centered).
In general, the presence of ferromagnetic properties is dependent on the presence of unpaired electrons in the electron configuration of the ground state of the metal or compound in question, which is essentially unique to transition metals and rare earths.
Ferromagnetism normally only occurs in the solid state because the Curie temperature of these materials is lower than the melting temperature. However, ferromagnetism has also been observed in a supercooled melt. Ferrofluids are suspensions of solid magnetic particles in a non-magnetic liquid.
Questions and Answers
Q: What is ferromagnetism?
A: Ferromagnetism is a property of materials such as iron, where they are naturally magnetic and are attracted to other magnets.
Q: What is the strongest type of magnetism?
A: Ferromagnetism is the strongest type of magnetism.
Q: In which technological objects is ferromagnetism used?
A: Ferromagnetism is used in everyday technological objects like credit cards and television screens.
Q: What are ferromagnetic materials?
A: Ferromagnetic materials are materials that have some magnetization on their own, meaning they are still magnetic even without any magnetic field from other objects at a temperature of absolute zero.
Q: What is the reason behind the magnetization of ferromagnetic materials?
A: The electrons in a ferromagnetic material have a spin, and the electrons like to have all the spins going one way, thus giving some magnetism to the material.
Q: What happens to magnetization as temperature is increased?
A: Usually, as the temperature is increased, magnetization goes down because the high temperature causes the electrons in the material to move around more, resulting in their spins not perfectly going in the same way anymore.
Q: What is the Curie temperature?
A: The Curie temperature is the point at which there is no magnetization anymore, and it changes among different materials.