Energy transition
This article deals in general with the transformation from fossil-nuclear power generation to an energy supply based on renewable energies.
For the concrete implementation in Germany and other states, see Energy transition by state
Energiewende is the German-language term for the transition from the unsustainable use of fossil fuels as well as nuclear energy to a sustainable energy supply using renewable energies. The term became culturally received after the 1980 book Energiewende - Wachstum und Wohlstand ohne Erdöl und Uran (Energy Turnaround - Growth and Prosperity without Oil and Uranium) by the Öko-Institut and has already been adopted as a loanword in other languages (for example, "The German Energiewende" or "A Energiewende alemã").
The aim of the energy transition is to minimize the ecological, social and health problems caused by the conventional energy industry and to fully internalize the external costs incurred in the process, which have so far hardly been priced into the energy market. In view of the fact that global warming is largely man-made, the decarbonization of the energy industry is particularly important today - by ending the use of fossil fuels such as oil, coal and natural gas. Likewise, the finite nature of fossil fuels and the dangers of nuclear energy represent important reasons for the energy transition. Solving the global energy problem is considered the central challenge of the 21st century.
The energy turnaround encompasses the three sectors of electricity, heat and mobility, as well as the perspective of moving away from fossil raw materials, for example in the production of plastics or fertilizers. A phase-out of coal and oil associated with the energy turnaround must also mean that substantial quantities of existing energy sources must remain in the ground. Key elements of the turnaround are the expansion of renewable energies, combined with the development of energy storage facilities, the increase in energy efficiency and the implementation of energy-saving measures. Renewable energies include bioenergy, geothermal energy, hydropower, ocean energy, solar energy (solar thermal, photovoltaics) and wind energy. Conceptually, sector coupling plays an important role, in particular the electrification of the heating sector by means of heat pumps and of transportation by means of electromobility.
The transition from conventional fuels to renewable energies is underway in many countries around the world. The concepts for the energy turnaround, as well as the technologies required for it, are well known. From a technical point of view, a complete global energy transition by 2030 is considered feasible. However, political and practical problems mean that implementation is only possible by 2050, with the lack of political will considered the biggest hurdle. Both on a global level and for Germany, studies concluded that energy costs in a renewable energy system would be at the same level as or cheaper than in a conventional fossil-nuclear energy system.
Denmark is considered a pioneer of the energy turnaround, already covering 30 % of its electricity requirements with wind energy in 2012. By 2050, Denmark aims to have a completely renewable energy supply in all three sectors. Also of significance is the German energy turnaround, which has met with worldwide approval and imitation, but also criticism and rejection. Although it is often wrongly associated with the second nuclear phase-out in 2011, the energy transition in Germany began as early as the 1980s with the expansion of renewables and the search for alternatives to nuclear power plants. While there is agreement in the scientific community about the fundamental need to expand renewable energies, increase energy efficiency and save energy, the concrete measures are often politically controversial. The public debate often reduces the concept of energy transition to the electricity sector, which in Germany only accounts for around 20 % of energy consumption. Likewise, the political and public debate often ignores the fact that, in addition to the expansion of renewable energies and the increase in energy efficiency, behavioral changes in the sense of energy sufficiency, i.e. energy conservation through a change in consumption habits, are also necessary for the success of the energy turnaround.
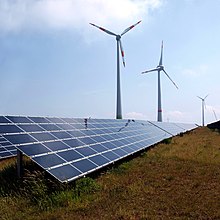

The integration of solar power and wind energy also requires new thinking: for example, load control on the consumer side and greater use of smart grids can make electricity demand more flexible.
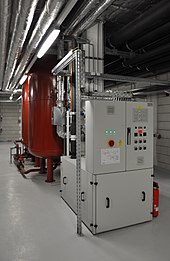

Electrically powered heat pump heating systems represent an important pillar of a future cross-sector networked flexible energy system.
.jpg)

New technologies such as the electric car and new concepts for its use such as car sharing are topics in the energy transition
Goals of the energy transition
Realization of a sustainable energy supply
→ Main article: Sustainability, sustainable energy supply and sustainable development
The core objective of the energy turnaround is the realization of a sustainable energy supply in the three sectors of electricity, heat and mobility. According to Alfred Voß, sustainable development is understood to be a way of life that enables "the needs of people living at present to be satisfied without compromising similar needs of people living in the future. [...] The preservation of the natural foundations of life, or in other words, the non-exceeding of the regenerative and assimilative capacity of natural material cycles, is thus an essential condition for sustainable development." The definition of sustainability in this context goes back to the Brundtland Commission, which coined this definition in 1987 and, in order to solve the environmental problems that had become urgent, called for economic growth in which "social and ecological aspects must be integrated spatially and temporally into the economic consideration".
According to a generally accepted definition, energy in a sustainable energy system "should be provided sufficiently and - according to human standards - for a long time in such a way that as many people as possible have the chance to live in dignity now and in the future, and substances that cannot be traced back to the transformation processes should be deposited in such a way that the foundations of human life are not destroyed now and in the future. "With the implementation of the sustainability thought an improvement in the sustainability triangle economy - society - ecology is to be produced thus and at the same time a global and generation-spreading solidarity to be reached. However, it is disputed in the academic sustainability discourse to what extent the sustainability triangle with equally weighted sectors is an appropriate premise, or whether ecological sustainability should not enjoy priority. Criticisms of the equal weighting are, in particular, the difficulty of optimizing the overall system as a result of conflicting goals between the three individual aspects and the equal weighting itself, since the preservation of livelihoods through ecological sustainability is a basic prerequisite for social and economic sustainability and must therefore be prioritized.
According to Eichelbrönner and Henssen, future energy systems are characterized by nine different requirements. It should be noted that the order does not imply any valuation, nor should any of these requirements be understood as a criterion for exclusion. The basic requirements of future energy systems are therefore:
- Provision of a sufficient amount of energy
- Demand-oriented quality of use and flexibility
- Energy Security
- Resource conservation
- Inherent low risk and fault tolerance
- Environmental compatibility
- International compatibility
- Social compatibility
- Low cost
The positive co-benefits resulting from the energy transition have increasingly found their way into political and scientific discourse in recent years. The Intergovernmental Panel on Climate Change (IPCC), for example, defines co-benefits as the positive additional benefits that result from the reduction of greenhouse gases.
Nuclear phase-out and climate protection
→ Main article: Nuclear phase-out and climate protection
So far, the goal of the energy transition has been reduced to the completion of the nuclear phase-out and climate protection; in some cases, all three terms are even used similarly or synonymously. Even though both nuclear phase-out and climate protection are important sub-goals of the energy transition, reducing the energy transition to these aspects is a misleading reduction. For example, it is comparatively easy to phase out nuclear energy by replacing it with fossil fuels, without requiring any further system restructuring. Climate protection, on the other hand, is in principle also possible by replacing today's fossil-fuel power plants with nuclear power plants and, with some restrictions, also with fossil-fuel power plants with carbon dioxide capture. However, this path would not be viable in the long term and would also involve major risks, which is why neither nuclear energy nor CCS technology are considered sustainable solution strategies for the current energy and environmental crisis. Although a switch to nuclear power plants and fossil power plants with CCS technology could avoid some of the environmental problems of the current energy system, the fundamental problem of finite fossil and nuclear energy sources would remain unsolved.
Since there is an approximately linear relationship between the cumulative total amount of greenhouse gases emitted and the resulting temperature increase, the cumulative amount of greenhouse gases emitted must be limited (i.e. capped) for effective climate protection. Therefore, only a part of the currently known fossil energy sources may be used. According to IPCC data, a maximum of between 870 and 1,240 gigatons (billion metric tons) of carbon dioxide may therefore be released in the period from 2011 to 2050 if the two-degree target is to be achieved with a probability of more than 50%. Converted to reserves, this means that in a global context, about one-third of oil reserves, half of natural gas reserves, and more than 80% of coal reserves may not be burned. The disproportion between known reserves of fossil energy and carbon that may still be burned results in the danger of the so-called carbon bubble bursting, which would represent a major loss of value for energy companies in the fossil energy business sector. At the same time, the exploration of new deposits that can no longer be exploited could prove to be a serious misinvestment in the long term. The value of fossil energy reserves is estimated at around $27 trillion. A business-as-usual policy, on the other hand, would result in the carbon budget for the two-degree target being depleted after 20 to 30 years, i.e. between 2035 and 2045.
To achieve the two-degree target agreed internationally in the ParisAgreement with a high probability, global carbon dioxide emissions of about 40 billion tons per year must be halved every decade. This will require, among other things, a doubling of renewable energy generation every 5-7 years by 2050. On the other hand, coal burning must be phased out entirely between 2030 and 2035 and oil burning between 2040 and 2045, and natural gas use must also be greatly reduced by 2050 even with the availability of carbon capture and storage technologies. Overall, humanity's entire energy base must be completely decarbonized. To achieve this, humanity has only a window of a few years to decades in which to minimize severe to catastrophic climate change impacts that may last thousands to tens of thousands of years.
Carl-Jochen Winter lists the following necessary ecological criteria for the transformation to a sustainable energy system:
- the avoidance of a nuclear or climatic catastrophe
- the way away from the use of energy raw materials and towards conversion technologies
- the optimization of mankind's material conversion system towards minimal material consumption and closed material cycles
- the use of solar forms of energy
Social and ethical goals
Apart from technical and ecological criteria, future energy systems must also fulfill social and ethical criteria in order to be considered sustainable. This includes, for example, finding a solution to the current lack of distributive justice in fossil energy use, both in terms of distributive justice today (e.g. between the inhabitants of rich industrialized countries and poor developing countries) and in terms of distributive justice across generations.
Improving public health
Another goal of the energy transition is to improve public health. Worldwide, around seven million people die prematurely each year from air pollution caused by short-lived climate-impacting pollutants such as methane, soot and ozone, which lead to respiratory and circulatory diseases. This effect will be exacerbated in the future by climate change and the heat island effect, especially in cities. In addition, air pollution exacerbates diseases such as asthma and cancer, increases stays in hospitals and intensive medical care, and increases the number of days of absence from work and school due to illness, which in turn has economic and social consequences for society and the national economy. Air pollution is caused in particular by the combustion of solid, liquid and gaseous fuels, which is why the solution to this problem can only be achieved through a fundamental change in the energy system.
A 2015 review article in The Lancet concluded that climate change has the potential to reverse the health gains made so far through economic development. Addressing it, on the other hand, could be the greatest opportunity of the 21st century for improving public health worldwide. For example, many climate change measures would also directly address health inequities, poverty, and global inequality, allowing countries to strengthen public health and well-being, not to mention reduce health care costs.
The main source of air pollution in industrialized countries is the combustion of coal in power plants and petroleum in the heating and transport sectors, while the use of natural gas contributes little to air pollution. Also an important source is the combustion of solid and liquid biomass such as wood or biofuels. Especially problematic are open hearths in buildings, as they are mostly wood-fired in most developing countries. Worldwide, about 2.8 billion people (41% of the world's population) use such stoves without a flue or chimney. The number of annual deaths due to this use is estimated at about 1.4 million.
It is considered almost certain that with large-scale deployment of renewable WWS energies (wind, hydro, and solar), a large number of health and environmental problems could be greatly mitigated or eliminated. For example, in addition to renewable energy deployment, electrification of the energy system with electric vehicles would also help reduce air pollution. Halving emissions of air pollutants by 2030 would avoid about 40 million premature deaths by 2040, according to Schmale et al. However, this would require much greater efforts than have been implemented to date; the measures implemented to date would only prevent about 2 million deaths. In addition, the avoidance of health damage can in some cases significantly overcompensate for the costs of an ambitious climate policy. Research for the U.S. found that the economic health benefits of replacing fossil energy exceeded wind energy subsidies in the form of the Production Tax Credit by about 60%. In addition, unlike climate change mitigation, which is global and only has a long-term effect, the health benefits from reduced air pollution are local and short-term.
Combating energy poverty in developing countries
→ Main article: Energy poverty (development policy)
Access to energy is currently very unevenly distributed around the world. This is accompanied by rampant energy poverty among broad sections of the population in many developing countries, which is further exacerbated by the growth of the emerging economies and the associated increase in global demand. With the accompanying rise in the price of fossil fuels, which are usually traded in hard currencies such as the U.S. dollar, access to energy sources, especially oil, is becoming increasingly difficult for developing countries that have only limited foreign currency reserves, further exacerbating poverty.
For example, around 2010, the G8 nations, which account for 12% of the world's population, were responsible for approximately 50% of global primary energy consumption, while the poorest 25% of the world's population accounted for only 3% of primary energy consumption. This is accompanied by other social problems: low access to energy correlates with poor access to food resources, high infant mortality and low life expectancy. However, if basic energy needs are met, which is the case from an annual per capita primary energy consumption of about 2.6 tons of oil equivalent, additional energy consumption does not improve these values. It is therefore considered an important goal of development policy to supply 1.2 billion people with electricity and 2.8 billion people with reliable and clean energy for cooking purposes.
Generational Justice
→ Main article: Generational justice
Furthermore, distributive justice between individual generations is of great importance. The use of fossil fuels by current or past generations affects two aspects of intergenerational justice: On the one hand, future generations can no longer use resources consumed by previous generations and are thus restricted in their right to development in the sense of strong sustainability. On the other hand, they are negatively affected by climate change as a result of the burning of fossil fuels, in that they have to cope with climate conditions that have changed to the negative and which they did not cause themselves. The present generation, on the other hand, benefits by not having to pay for the ecological and economic consequences of its use, but can pass on the solution to the problems it has caused to future generations. Generational justice, on the other hand, presupposes that each generation must be free to decide for itself how it wants to produce and distribute goods. This freedom of future generations is, however, inadmissibly severely restricted by the current generation through actions that cannot be reversed in the near future; for example, through man-made climate change and its consequences, the overuse of raw materials and (fossil) energy sources, or the destruction of animal and plant species. The main difficulty of this conflict is the fact that future generations cannot participate in its management, but this task can only be performed by the state or its institutions.
Other aspects
In addition, a number of other aspects for the implementation of the Energiewende are mentioned in the scientific, public and political discourse; for example, an expert survey on the German Energiewende revealed a total of 14 different objectives. These goals are, among others, political, social, economic or ecological in nature; some examples are listed in the following by way of highlights.
- Democratization of production and distribution structures, realizable e.g. in the form of energy cooperatives or energy-autonomous regions
- Reduction of the economic risks of an energy shortage or an energy crisis (e.g. oil crisis) through virtually unlimited primary energy
- Avoidance of military conflicts over energy resources
- Economic benefits from a cheaper energy supply in the long term
- Economic value creation through production and export of climate protection technologies
- Creation of new jobs, as the use of renewable energies is more labor-intensive than conventional energy production
- Increasing domestic value creation by reducing energy imports
- Reduction of water consumption by reducing the cooling water consumption of conventional power plants (currently in Germany about 0.9 to 1.33 m³/MWh for coal-fired power plants and 1.44 to 2.12 m³/MWh for nuclear power plants)
- Energy savings due to lower power plant own requirements of regenerative compared to fossil power plants
- Positive effects on the labor market: Depending on the country and expansion scenario, replacing coal-fired power plants with renewables can more than double the number of jobs, per installed MW capacity.
- Improved security of energy supply in regions previously only partially electrified, also improved quality of education through stable energy supply.

Like the nuclear phase-out, climate protection is an important goal of the energy transition, but there are many more goals.
_jobs.jpg)

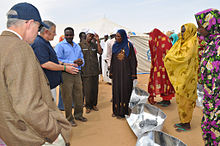

Solar stoves can help alleviate the energy poverty that often exists in many developing countries with high levels of direct solar radiation.
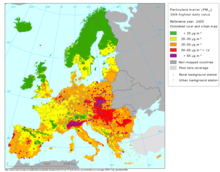

particulate matter (PM10) pollution in Europe.
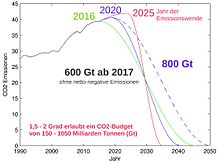

Necessary emission reduction pathways to meet the two-degree target agreed in the Paris Agreement without negative emissions, depending on emissions peak
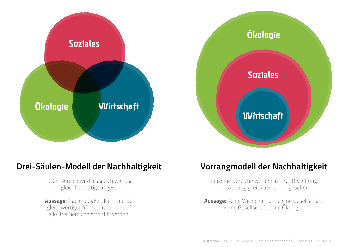

Different models of the sustainability concept: on the left, the three-pillar model, which gives equal weight to ecology, economy and social issues; on the right, the priority model, which postulates the dependence of social sustainability and economic sustainability on ecological sustainability.
Economic consideration
→ Main article: Economic efficiency of renewable energies
Economic forecasts of the transformation of the energy system are subject to considerable uncertainty, since the transformation of the energy system is a process that extends over several decades, and at the same time the forecast must take into account a number of technological, economic and social variables whose development can only be estimated in part. These include, for example, the development of the electricity production costs of conventional and renewable generators, the price trend of energy raw materials and possibly pollution rights, changes in demographics and energy consumption, or the selected future energy infrastructure (more centralized or more decentralized?). Additional cost factors compared to conventional energy supply result from the necessary reconstruction of the energy infrastructure in the context of the energy transition, such as grid expansion and the integration of energy storage systems, while the avoidance of greenhouse gas emissions, climate change impacts and health damage due to pollution caused by the combustion of fossil fuels results in economic cost savings. Depending on the weighting of the different factors, the results can vary and are sometimes controversial, especially in the public debate in Germany.
Economic consideration
External costs by energy source in Germany (2007) | |
Energy source | ct/kWh |
Lignite | 8,7 |
Hard coal | 6,8 |
Heating oil | 6,1 |
Natural gas | 3,9 |
Photovoltaics | 0,8 |
Hydropower | 0,4 |
Wind energy | 0,1 |
Electricity mix 2005 | 5,8 |
Power generation incurs both internal (i.e., business) and external, economic costs. While internal costs mainly consist of the construction, operation and dismantling of power plants as well as fuel procurement, which are mostly subject to market mechanisms, external costs manifest themselves primarily in the form of environmental pollution, health and climate damage, which are borne not by the polluters but by the general public. The actual full costs of power generation are therefore difficult to determine; in some cases, the social and environmental costs of conventional power generation even exceed the retail prices paid by electricity consumers.
A number of studies on external costs are available for the USA. Shindell, for example, puts the environmental damage from electricity production in the U.S. at $330-970 billion per year, most of which is attributable to the burning of fossil fuels. Machol and Rizk concluded that the health damage attributable to fossil fuel combustion alone causes total economic damage of approximately $362-887 billion per year. This would result in consequential health costs of 14 to 35 U.S. cents/kWh, which significantly exceeds retail electricity prices there. Jacobson et al., on the other hand, examined the external costs of the entire energy sector (electricity, heat, and transport) and quantified the external costs saved in the event of a complete energy transition by 2050. According to this, a switch by the U.S. to a 100% renewable energy supply in 2050 would save about $600 billion annually in health costs and about $3.3 trillion in climate damage compared to the status quo.
If a full-cost calculation is made that also includes external costs for the respective technologies, many renewable energies are already cheaper than conventionally generated electricity. Although they also incur external costs, these are significantly lower than for fossil fuels. However, since the external costs of conventional energy generation are not yet reflected in the prices of fossil energy sources, in practice there is a market failure in favor of the conventional energy industry. Under the current economic conditions, the market principle thus leads to a suboptimal use of energy resources: fossil energy sources appear cheaper than they are in economic terms because external costs are not taken into account. However, if the market is to find the most economically efficient production method, as liberalization aims to do, it is imperative to avoid all factors that distort competition and to establish true costs by internalizing all external factors. If this is not done, the efficiency advantages of a liberalized market can be nullified by negative effects on the environment. Possibilities for establishing this cost truth with regard to global warming are incentive taxes such as a CO2 tax or emissions trading.
So far (April 2014), only a small part of these external effects has been internalized, and full internalization is not foreseeable. For example, the annual report on energy consumption in Germany in 2013 by AG Energiebilanzen concludes that "the incentives for emission-reducing behavior intended by emissions trading are not to be expected at such certificate prices [of approx. 5 euros/ton]". The lack of internalization of external costs is seen as a decisive obstacle to the progress of the energy transition.
To this end, the cost of electricity is heavily distorted by subsidies for individual technologies, with conventional energy sources receiving subsidies several times higher than renewable energies, which thus lose competitiveness. In 2011, fossil fuels were subsidized to the tune of $523 billion worldwide, while renewables were subsidized to the tune of about $100 billion. Including external costs, fossil energy subsidies that year amounted to about $1.9 trillion, according to Jiang and Lin. The International Monetary Fund, on the other hand, cites higher figures. According to them, when the economic costs of environmental and health damage are included, subsidies totaled $4.2 trillion in 2011 and $4.9 trillion in 2013. In 2015, subsidies are estimated to be $5.3 trillion, equivalent to 6.5 percent of world gross national product, higher than global spending in the health sector. At the same time, these subsidies for oil, coal, and gas would keep energy prices artificially low, slow the expansion of renewable energy, and increase climate-damaging emissions by 17%. Based on these figures, the production of a ton of carbon dioxide in the energy sector was subsidized by more than $150 worldwide in 2013.
Business management consideration
Energy source | Electricity production costs in ct/kWh | ||
Data origin: Fraunhofer ISE 2018 | |||
Lignite | 4,59–7,98 | ||
Hard coal | 6,27–9,86 | ||
Natural gas C&I | 7,78–9,96 | ||
Natural gas turbine power plant | 11,03–21,94 | ||
Wind/Onshore | 3,99–8,23 | ||
Wind/Offshore | 7,49–13,79 | ||
Biogas (without heat extraction) | 10,14–14,74 | ||
Photovoltaic small system roof | 7,23–11,54 | ||
Large photovoltaic system roof | 4,95–8,46 | ||
Large-scale photovoltaic power plant open space | 3,71–6,77 |
Ignoring the external costs of conventional and alternative energy generation and considering only the business costs, most renewable energies currently (2018) have roughly similar electricity production costs in Germany as conventional energies. An exhibition of the LCOE of individual technologies can be found in the table on the right.
Particularly in the case of photovoltaics and onshore wind energy, there has been a continuous decline in the cost of electricity. This has been triggered by a strong expansion of production and the resulting economies of scale, strong competition and further technical developments ('learning curve'), e.g. by improving efficiency. Further decreases in electricity production costs are expected, while the opposite effect is observed for conventional energies.
However, a comparison of fossil and renewable energy systems must also take into account the additional costs of grid expansion and electricity storage that would be incurred in a renewable energy system. Assuming that electricity is distributed primarily through a cross-state smart supergrid to exchange regional variations, Jacobson and Delucchi conclude that these additional costs are unlikely to exceed 2 U.S. cents/kWh. Therefore, in the face of further long-term increases in fossil fuel costs, it is assumed that, in the long run, energy costs in a renewable energy system will be at the same level as or cheaper than in a fossil-nuclear energy system.
Already since the beginning of electricity production, hydropower has been an integral part of the electricity mix due to its low production costs; for a few years now, wind power plants at good onshore locations have also been competitive with conventional power plants without subsidies. In the future, it is expected that wind power will also be competitive with coal-fired power plants at poorer sites and will become the cheapest form of electricity production. In addition, more and more older wind turbines are reaching an age when they are depreciated or fall out of statutory support and can then produce electricity more cheaply for this reason. However, this constellation is only possible if the service life of a wind turbine exceeds the subsidy period.
Globally, the LCOE of wind and photovoltaic plants in particular has also fallen sharply, a trend that Walwyn and Brent expect to continue until at least 2030. In the best locations, the cost of electricity from wind turbines is now $40-50/MWh (€33.5-41.8/MWh), although this is highly dependent on site quality and financing conditions. In the US, onshore wind is already the second cheapest power plant after gas-fired CCGT. The situation is similar for photovoltaics, where module prices fell by 60% between January 2011 and December 2012, and are expected to fall further. Overall, module prices fell from $6-7/watt in 2000 to $0.5-0.6/watt in 2013. In photovoltaics, the industry is now talking about Swanson's Law, which states that the price of solar modules falls by 20% as the number of modules shipped doubles.
Photovoltaic systems, solar collectors and, to some extent, wind turbines and biogas plants can be installed as small-scale power plants directly at the end consumer. Because of the resulting elimination of transport costs and taxes due to self-consumption, such power plants do not have to compete or only partially compete with wholesale prices; instead, profitability is determined by end-customer prices for electricity and, if applicable, heat. Thus, in many countries, grid parity for photovoltaics was already achieved a few years ago for private households, municipalities and most commercial enterprises, even though the electricity production costs are still higher than those of conventional power plants. In Germany, grid parity for photovoltaic electricity was achieved in 2012. Falling prices of solar power are expected to result in strong changes in the power sector in the future.
In many countries around the world, photovoltaic island systems with battery storage are also the most favorable form of electricity supply for individual buildings or settlements in rural areas. From an economic as well as an ecological point of view, such systems perform better than the alternatives of diesel generators or electrification through connection to a power grid.
Questions and Answers
Q: What is energy transition?
A: Energy transition, also known as Energiewende in German, refers to the process of transitioning from non-renewable energy sources like nuclear and coal towards a sustainable economy based on renewable energy, energy efficiency, and sustainable development.
Q: Which countries have committed to energy transition?
A: Apart from Germany, several other countries have committed to energy transition as a means of achieving sustainable economies. Some of these countries include Denmark, Sweden, Portugal, and Costa Rica.
Q: What is the final goal of energy transition?
A: The final goal of energy transition is the complete abolishment of non-renewable energy sources like nuclear and coal in favor of renewable energy sources like wind, solar, biomass, and hydropower.
Q: What are the benefits of energy transition?
A: Energy transition offers numerous benefits, including reducing greenhouse gas emissions, creating new job opportunities, stabilizing energy prices, improving energy security, and enhancing public health and safety.
Q: What challenges does energy transition face?
A: Energy transition faces challenges such as high upfront costs, inadequate policy support, insufficient investment in research and development, market barriers, and technical limitations.
Q: What role can individuals play in energy transition?
A: Individuals can contribute to energy transition by adopting energy-efficient practices in their homes and workplaces, investing in renewable energy sources, and advocating for policy changes that support a transition to sustainable economies.
Q: How can governments support energy transition?
A: Governments can support energy transition by implementing policies and regulations that incentivize the transition towards renewable energy sources, providing funding for research and development, implementing energy-efficient building codes, and investing in public transportation and infrastructure.