Electric charge
The electric charge (amount of electricity) is a physical quantity, which is connected with the matter, like e.g. also the mass. It determines the electromagnetic interaction, i.e. how matter reacts to and causes electric and magnetic fields. Its formula symbol or
derived from the Latin word 'quantum'. In the International System of Units, the charge is given in the derived unit coulomb (= ampere-second).
Electric charge is one of several types of charge that occur in elementary particles. Elementary particles can carry positive, negative, or no electric charge. The charge of free particles is always an integer multiple of the elementary charge , which is
is. The charge of the electron is negative:
, that of the proton positive:
. For composite particles such as atoms, the individual charges add up. If the total charge is zero, the composite particle is called neutral. In a closed system, the total charge is invariant (conservation of charge). The charge of a particle is the same in every reference frame within the framework of the relativity theory, thus a Lorentz invariant.
Electrostatics considers charges at rest and purely electric fields. The Coulomb force between positively and negatively charged bodies is attractive, between charges of the same name repulsive. Extended physical systems always contain approximately equal numbers of positive and negative elementary charges. Even relatively small excess charges can cause considerable field strengths and forces (example thunderstorm).
Moving electric charges form an electric current. They generate electromagnetic fields and their motion is influenced by them. This behavior is described in classical electrodynamics.
At the microscopic level, the electromagnetic interaction between charges, together with quantum mechanical effects such as the Pauli principle, is the cause of the cohesion and structure of matter.
Everyday Observations
Almost all physical phenomena observable in everyday life are based on one of two fundamental interactions, gravity or the interaction of electric charges. Electrical forces between the electron shells of atoms are essential to explain chemical processes and, in general, the experiential properties of matter - even though quantum mechanical properties such as spin must often be taken into account to fully understand them.
Charged objects can also make themselves felt through forces. If packaging material, for example small polystyrene parts, seem to move by themselves, the electrostatic attraction or repulsion of charged particles is behind it.
An impressive consequence of electrical charges due to frictional electricity is thunderstorm lightning. Air is normally an insulator, but at high voltages, breakdown occurs. In lightning, an abrupt charge equalization occurs between differently charged areas in the thunderstorm cell or, more rarely, between an area in the thunderstorm cell and the ground. Small sparks accompanied by a crackling sound can also occur when putting on or taking off clothing or when combing.
Humans do not have a specific sensory organ for electrical charge. He can only perceive it indirectly when the current through the body or the voltage dropping across the skin resistance reaches the threshold value of the action potential of the neurons. A slight electric shock is felt, as mentioned above, when taking off clothes, or when walking across a carpeted floor and then touching a door handle. Electric current can cause pulling pain in the dental nerve when electrochemically dissimilar metals (for example, aluminum foil and amalgam) are in contact in the mouth and a local element is formed. Similarly, a tingling sensation in the tongue is caused by current flow when one touches both poles of a suitable battery with a wet tongue.
But also the fact that solid matter repels each other and one cannot reach through solid matter is due to the Coulomb force, which in turn depends on the electric charge.
.jpg)

Lightning between clouds
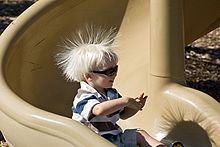

Erected hair after charging with frictional electricity
History
Naming
Probably already around 550 BC, Thales of Miletus in ancient Greece conducted experiments in which the forces emanating from electrical charges were observed. For example, an attractive force on bird feathers or hair emanating from a piece of amber (Greek ηλεκτρόν - pronounced elektron) was detected after the amber had been rubbed against a dry coat.
The court physician of Queen Elizabeth I, William Gilbert, continued the work of Petrus Peregrinus from the 13th century and found that other substances can also be electrified by friction. He listed in his 1600 book De Magnete, Magnetisque Corporibus, et de Magno Magnete Tellure (German roughly: On the Magnet, Magnetic Bodies, and the Great Magnet Earth), he introduced the term "electrica," borrowed from Neo-Latin, for the phenomena he discovered in connection with amber. Later this term became as electron the designation for the carrier of the negative elementary charge, the electron so designated in 1891 by George Johnstone Stoney and proved in 1897 by Joseph John Thomson (also the rubbed amber takes a negative charge).
One or two types of cargo
William Gilbert is considered the founder of electricity theory because of his work. He was the first to distinguish between electrical and magnetic attraction. His explanation for the attraction of a rubbed amber to other bodies was that he assumed an "imponderable" (i.e. imponderably light) fluid in all bodies that could be influenced by friction, which escaped through the heat during friction and surrounded the body like a cloud of vapor. Other substances would be attracted to this haze when it penetrated, analogous to the attraction of a stone by the earth. In Gilbert's fluidum theory or fluidum hypothesis, from today's point of view, something of the modern concept of the field sounds. However, the differences are considerable, especially because the haze consists of leaked fluidum. Otto von Guericke dealt with static electricity in his late work, but little of his results has survived. In 1672, he invented a simple electrifying machine, with the help of which he was able to observe a whole series of phenomena, such as the influence, the conduction of electric charge, the luminous effect (electroluminescence) and the fact that two bodies electrified with the same name repel each other. Until then, only the attraction effect of electricity was known; Gilbert's attempt to explain the one fluid was now no longer sufficient.
In 1733, Charles du Fay realized during experiments with frictional electricity that the two types of electricity could neutralize each other. He designated the types of electricity as glass electricity (French électricité vitreuse) and resin electricity (French électricité résineuse). In today's terminology, glass electricity corresponds to the positive charge. From these experiments, Jean-Antoine Nollet developed the so-called "two-fluid theory" or dualistic theory, as also advocated by Robert Symmer. According to this theory, the two kinds of electricity surround the electrified bodies as "two fluids" (the effluvium and the affluvium). According to this theory, an electrically neutral body contains the same amount of both fluids. Then, when two bodies touch intimately, positive fluid passes from one body to the other, while the other body gives the same amount of negative fluid to the first. This way of speaking shaped thinking about the nature of electricity in the 18th century and still lives on today in the "two types of charge" (positive charge vs. negative charge).
In the book Experiments and Observations on Electricity written by Benjamin Franklin - on the subject of electrical phenomena - he coined the term charge. Before, it was necessary to speak of "bodies which have been put into an electric state", Franklin introduced a view as with the loaded and unloaded account, where redistributions occurred by friction. William Watson came to a similar assessment at the same time. Thus, according to this unitary theory or single fluid theory, the only fluid is present in a certain normal amount on bodies that appear electrically neutral. When two bodies rub against each other, a certain amount of this fluid then passes from one body to the other, so that the change in the location of the fluid causes one body to be positively charged and the other to be negatively charged to the same extent. Franklin could not explain with his view why two bodies equally emptied of charge repel each other, only Franz Ulrich Theodor Aepinus remedied this deficiency. In today's parlance, he saw the particles of matter in a certain ionized state when the charges were removed.
Franklin's assumption that the electricity of the glass exists and the resin electricity is a deficiency, and that when charged and uncharged bodies touch, the electricity always flows in one direction only, suggested that - in today's terminology - the positive charges are always moving. Probably Franklin was led to this assumption by the kind of observable luminous phenomena in his experiments with charged metal points.
With this new theory of electricity as "a fluid" the idea of conservation of charge was helped to a breakthrough. Charges are not created by friction, but only separated from each other. Since the direction of force between two charges can be described simply by the sign of the charges involved with the help of the two-fluid model, Charles Augustin de Coulomb adopted the dualistic model of "two fluids" and based it on the existence of two types of charges. From today's point of view, the same result can be obtained with both models.
In German-speaking countries, Franklin's method of designation was probably spread primarily by Leonhard Euler and Georg Christoph Lichtenberg.
Quantitative experiments
In 1675, Robert Boyle established that electrical attraction or repulsion also occurs through a vacuum, and Francis Hauksbee deepened these investigations using electrical luminous phenomena in a vacuum. In 1729, Stephen Gray divided materials into electrically conductive and electrically insulating and demonstrated that the human body could also conduct electricity.
In the last quarter of the 18th century, the focus of the now very popular study of electricity (after an impressive experimental device had been found in the Leiden bottle) shifted to quantitative studies of electrostatics. Particular contributions to the research were made by Joseph Priestley and Charles Augustin de Coulomb. Coulomb published Coulomb's Law in 1785, which states that the magnitude of this force between two charged spheres is proportional to the product of the two quantities of charge and inversely proportional to the square of the distance between the centers of the spheres. Depending on the sign of the charges, the force acts attractively or repulsively in the direction of the straight lines connecting the centers.
Faraday's laws, formulated by Michael Faraday in 1832, establish a relationship between electric charge flowed and mass transfer (amount of material deposited at the electrodes) during electrolysis. In a lecture delivered to the Royal Society in 1833, Faraday proved that what had hitherto been conceived of as "different types of electricity" - "static" (or "ordinary"), "atmospheric", "physiological" (or "animal"), "Voltaic" (or "touch") and "thermoelectric" - were in fact only different aspects of the one physical principle he called "magneto-electricity". Thus, it was also clear that electric charge was the basic property of matter for all these phenomena. An important contribution of Michael Faraday to the theory of electricity was the systematic introduction of the field concept to describe electric and magnetic phenomena.
In 1873, Frederick Guthrie discovered that a positively charged electroscope discharges when a grounded, glowing piece of metal is brought near it. Nothing happens when the electroscope is negatively charged, from which it followed that glowing metal can only give off negative charge and this electric current can only flow in one direction. Thomas Edison rediscovered this phenomenon in 1880 during experiments with incandescent lamps and applied for a patent for an application based on it in 1883. The "incandescent electric effect" is called the Edison-Richardson effect after Edison and Richardson, who was awarded the Nobel Prize in 1928 for the explanation.
In 1897, Joseph John Thomson was able to prove that cathode rays consist of electrons. Using a greatly improved vacuum, he was able to determine the charge-to-mass ratio for them. Thomson assumed that the electrons were already present in the atoms of the cathode, and in 1903 he established an atomic model for the first time, which attributed an internal structure to the atoms.
The discrete character of electric charge, which had already been predicted in the 19th century by Faraday in the course of his electrolysis experiments, was confirmed in 1910 by Robert Andrews Millikan in the so-called Millikan experiment. In this experiment it was proved that charged oil droplets are always charged with an integer multiple of the elementary charge, it also provided a useful numerical value for the size of the elementary charge.
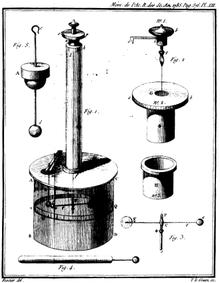

Coulomb constructed a torsion balance that allowed measurement of the force acting between charges.
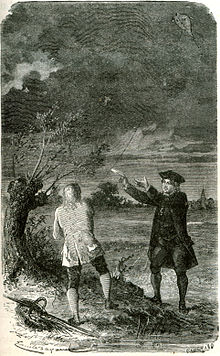

Benjamin Franklin's kite experiment during a thunderstorm: He was the first to interpret lightning as gigantic sparks.
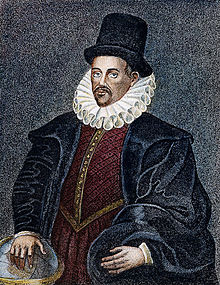

William Gilbert is considered the founder of the theory of electricity.
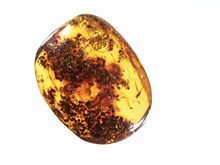

The term "electricity" is based on the Greek word for amber.
Properties of the electric charge
Total charge
The electric charge can assume positive or negative values. One often speaks of two types of electric charges. For example an electron or a muon has the charge -1 e, a positron or a proton the charge +1 e.
A particle and its antiparticle have exactly the opposite same amount of charge. For example, the antiproton, antiparticle of the proton, carries the charge -1 e.
The absolute charge of a body or a quantity of material is the sum of all elementary charges contained. The terms total charge, net charge or excess charge are also used for this. The meaning of this term is based on the fact that the electrical effects of positive and negative charges cancel each other out if their mutual distance is negligibly small compared to the distance to the point of action. Thus, at distances of a few nanometers, the lithium ion shown acts as a single charge carrier with a single positive charge, and is written as such, Li+. The cancellation also works exactly with hundreds of billions of charge carriers, as in the case of Millikan's oil droplets already described.
On the one hand, a particle that carries no charge (for example a neutron, gray in the lithium atom picture) is called electrically neutral. On the other hand, a body is also called neutral which carries an equal number of positive and negative elementary charges, for example a helium atom with two protons and two electrons.
Charge separation occurs when charges of one sign predominate in certain areas of space, i.e. the absolute charge there is not zero. In the case of charge separation within a body or component, it is therefore not sufficient to specify the total charge. For example, the total charge of both the charged and the uncharged capacitor can be zero. However, while the plates of the uncharged capacitor are each electrically neutral, the plates of the charged capacitor carry opposite excess charges which generate an electric field, especially between the plates. Oil droplets present there, for example, are polarized.
Charge conservation
→ Main article: Charge conservation
Charge conservation is the phenomenon that in any closed system the amount of electric charge present remains constant in time. This phenomenon has consequences: If matter is created from electromagnetic radiation or photons, then this must happen in such a way that no charge is generated. Therefore, during the pair formation, for example, an electron and its antiparticle, the positron, are created at the same time. Thus the produced total charge is zero, the charge quantity remains. Likewise it behaves with the reversal of this process, the pair annihilation of a particle-antiparticle pair, with which the annihilated total charge is also zero.
As with any fundamental physical conservation law, the law of conservation of electric charge is based on observations and experiments. So far, all relevant experiments have confirmed the conservation of electric charge - in some cases with very high accuracy. In the formal theoretical description of electrodynamics, charge conservation is expressed by a continuity equation, which is a corollary of Maxwell's equations (see the section on charge and electric current). A more abstract property of electrodynamics is its invariance (often called symmetry) under gauge transformations, which gives rise to quantum electrodynamics as a gauge theory. According to Noether's theorem, the invariance of electrodynamics under gauge transformations is also connected with the electric charge as a conservation quantity.
In apparent contradiction to the conservation of charge is the expression of a charge generation or charge. However, this means a local accumulation of charges of one sign, i.e. actually a charge separation (and no generation).
Charging
To charge (in the sense of an excess charge) a previously neutral body, it must take up or give off charge carriers. But also in the case of an uneven charge distribution in an overall neutral body, one speaks of "charging". This occurs, for example, due to an applied electric field or due to movements on a molecular scale. In the case of a polarized material, the charge is bound; in the case of influence, "freely moving" charge carriers are displaced in a conductor.
A mechanism for the separation of charges known from everyday life is friction. For example, if you rub a balloon against a sweater, electrons are transferred from one material to the other, separating electrons and the atomic hull that remains behind. Such frictional electricity is a special case of touch electricity. The ribbon generator uses both touch electricity and influence.
In batteries and accumulators, chemical reactions are used to redistribute a large amount of charge carriers (electrons or ions). As with the capacitor, the total charge remains zero. Unlike the capacitor, however, the voltage does not increase almost linearly, but remains approximately constant. For this reason, the capacity as an energy store in the case of the capacitor is expressed in farads (= coulombs per volt), while the capacity of a battery is characterized as the amount of charge - in ampere-hours, where 1 ampere-hour is equal to 3600 coulombs.
Charge separation can also be caused by electromagnetic waves, for example light: If light of sufficiently high frequency is allowed to strike a metal surface and a second metal plate is placed nearby in a vacuum, a charge difference is created between them because the light causes electrons to be released from the first plate, some of which move to the second plate (external photoelectric effect).
Relativistic invariant
The charge of a body is not only a conservation quantity, but also independent of its velocity. That is, electric charge is a relativistic invariant; the total charge of an object is not changed by length contraction. This property is shared by charge with the invariant mass of a system, but distinguishes it from energy, for example. From this example, one can see that relativistic invariance is not self-evident even for conservation quantities, but is an additional property.
At the computational level, the relativistic invariance of the charge can be understood by taking it as a volume integral over the charge density ρ
Under a Lorentz transformation, the charge density transforms like the time component of a four-vector, thus undergoing a change analogous to time ; the volume element on the other hand, undergoes a Lorentz contraction. These two effects exactly cancel each other out, so that the charge itself remains unchanged.
Interference experiments (for example by Claus Jönsson) with electrons of different velocities show directly that their charge is independent of the velocity. Moreover, otherwise the charge of a solid would have to change with temperature change, because the velocity of its constituents has increased due to the increased thermal energy, but the electrons acquire on average a much greater velocity than the more massive positive atomic nuclei. Also, hydrogen molecules and helium atoms (both contain two protons and two electrons) are electrically neutral, although the relative velocities of their constituents differ significantly.
Quantum character
See also: Millikan experiment
Electrically charged matter cannot carry arbitrary amounts of charge. The charges of all known elementary particles have been measured experimentally with the result that all leptons and their antiparticles always carry integer multiples of the elementary charge Of the building blocks of atoms, the proton and electron carry the charge
respectively.
the neutron carries no (electric) charge. Although quarks carry the charge
or
, but quarks never appear freely (see confinement), but always only in bound states, the hadrons, which in turn always carry integer multiples of the elementary charge. Thus all freely appearing particles carry integer multiples of the elementary charge.
This is theoretically justified in the electroweak model by attributing the electric charge to the weak hypercharge and the weak isospin. However, why the weak hypercharge and the weak isospin only assume certain values cannot be explained by the model. Therefore, the "cause" of the observed quantization of the charge is also unexplained so far; according to John David Jackson, it belongs to the greatest mysteries of physics. According to Paul Dirac's consideration of a magnetic monopole, the existence of such a particle - and thus of magnetic charges - would unavoidably lead the charge quantization back to the quantization of angular momentum. Considerations from quantum field theory lead the charge quantization back to the demand for anomaly freedom of the standard model.
Outside atomic structures, it is usually permissible to regard charge as a continuous quantity. Even a tiny current of 1 nanoampere means a directional charge transport of about six billion electrons per second. Thus, individual elementary charges are not detectable in most aspects of electrical engineering. An exception is the "shot noise".
Electric charge in quantum field theory
In the framework of quantum field theory, the elementary charge is the coupling constant of electromagnetic interaction. However, from the point of view of the renormalization group, the coupling constants of quantum field theories are not constants, but depend on the energy scale. The elementary charge also depends on the energy scale, becoming larger as the energy increases. This means that at very high energies, the interaction between charged particles is stronger. As a result, at high energies, particle reactions due to the electromagnetic interaction are more likely to occur. For example, the probability that an electron-positron pair will be formed when two electrons collide increases with the energy of the collision.
The electroweak model states that both electromagnetism and the weak interaction are only effective interactions at low energies, which result from a spontaneous symmetry breaking by means of the Higgs mechanism. The electromagnetic interaction is thereby described by the unbroken part of the symmetry, so that an electric charge can be defined according to the Fabri-Picasso theorem. At higher energies, according to the model, two other interactions take the place of electromagnetism and the weak interaction and the electric charge is replaced by the weak hypercharge and the weak isospin. According to this, the electric charge can be considered in a certain sense as composed of these two charge types.
The symmetry of positive and negative charge is important for quantum field theory. The transformation which reverses all signs of the electric charges in a particle system is called C. Other important transformations in the following are P, the point reflection of space at zero, and T the reversal of time direction. The CPT theorem, a fundamental statement about all quantum field theories, states that scattering processes are exactly the same when all these three transformations are applied to the system. This is generally not true for the individual transformations, since there are parity-violating and CP-violating processes. However, electrodynamics is both P and C conserving.
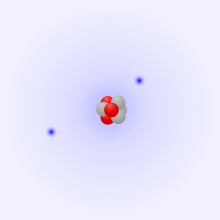

Li+ with three protons (red) and two electrons (blue). In units of the elementary charge, the total charge is (+3) + (-2) = +1.
Electric charge measurement
The charge quantity of 1 coulomb corresponds to about 6.24 - 1018 elementary charges. For the determination of total charges, therefore, it is usually not possible to simply count the charge carriers.
Indirectly, the amount of charge flowing out or in can be determined by measuring the current strength: If a current of constant strength flows during time
, it transports the charge
. In general, the charge that has flowed into or through a body is the integral of the electric current over time. If the discharge time is short compared to the period of oscillation of a ballistic galvanometer, the charge can be read directly as the amplitude of the impinged oscillation.
In principle, one can also determine the value of a charge measuring the magnitude
the force on a charged test body in an electric field of known field strength
The definition of the field strength provides the relation
This method is subject to severe limitations: The test body must be small, movable and electrically very well insulated. Its charge must not noticeably influence the electric field, but this is difficult to verify. Therefore, the charge should be small - but then the force is also difficult to measure.
The listed disadvantages do not possess another method, it succeeds also with quite large charges. The basis is the relation between the capacitance a capacitor and the electric voltage
:
A capacitor of known capacity is charged with the charge to be measured and then its voltage is measured. However, this measurement must be made with high impedance, i.e. in such a way that it takes only a negligible amount of the stored charge from the capacitor. This is done with an electroscope or, better, with an impedance transformer. However, with this method, the capacitance of the charge source must be known, since some of the charge remains there. The voltageless measurement with an integrator (without input resistance also called charge amplifier) avoids this problem.
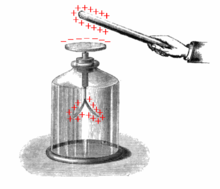

Electroscope in textbook from 1881
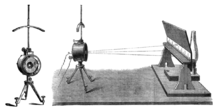

Thompson's mirror galvanometer
Questions and Answers
Q: What is electric charge?
A: Electric charge is a basic property of electrons, protons and other subatomic particles. Electrons are negatively charged while protons are positively charged. Things that have the same charge push each other away (they repel each other).
Q: Who discovered the Law of Charges?
A: The Law of Charges was discovered by Charles-Augustin de Coulomb.
Q: What does Coulomb's Law describe?
A: Coulomb's Law describes how strongly charges pull and push on each other.
Q: How do neutral objects differ from those with positive or negative charges?
A: Objects that have equal numbers of electrons and protons are neutral, while things that have more electrons than protons are negatively charged, and things with fewer electrons than protons are positively charged.
Q: What happens when two objects with different charges come into contact?
A: When two objects with different charges come into contact, they will attract each other - if possible, the one with too many electrons will give enough electrons to match the number of protons in the one that has too many protons for its load of electrons. If there are just enough electrons to match the extra protons, then the two things will not attract each other anymore.
Q: How does an electrical current form?
A: When electrons move from a place where there are too many to a place where there are too few, that is called an electrical current.
Q: Why can electric shocks be dangerous in some cases but not others?
A: Electric shocks felt when one gets a shock from a doorknob or other object usually is between 25 thousand and 30 thousand volts; however, because the electric current only flows briefly, it does not cause physical harm. On the other hand, when clouds gain electrical charges they have even higher voltages and amperage (the number of electrons that will flow in the lightning strike) can be very high - this means that if those high-voltage/high-amperage electrons go through a person it can burn or kill them.