Climate variability and change
This article deals mainly with natural climate factors in temporal relation to Earth's history.
For changes in the global climate system due to human influences since industrialization, see Global warming. For other meanings, see Climate change (disambiguation).
Climate change, also climate variability, climate change or climate change, is a globally occurring change in the climate on Earth or Earth-like planets/moons that have an atmosphere. The cooling or warming associated with climate change can occur over varying periods of time. An important distinction is made between those weather patterns that occur within the context of a climate state or zone, and climate change itself, which increases or decreases the probability of certain weather patterns occurring.
The current (anthropogenic) global warming, which is mainly caused by humans (see there), is an example of a climate change that is proceeding very rapidly but is not yet complete. The term climate change is often used as a synonym for this in public discussion (but then as "climate change"). The ecological and social crisis caused or predicted by current climate change is also referred to as the "climate crisis".
Climate change on a global scale is essentially due to a change in radiative forcing, which shifts the Earth's climate system from a stable thermal-radiative equilibrium to a new equilibrium. Radiative forcing results from atmospheric concentrations of greenhouse gases such as carbon dioxide (CO2), methane (CH4) and water vapour (H2O), from varying solar irradiance due to Milanković cycles, and from the reflectivity (albedo) of the Earth's surface including the oceans. The climate state during the last millions of years was that of an ice age and was predominantly controlled by the Milanković cycles, which significantly changed the solar radiation over several tens of thousands of years, thus providing the impetus for the alternation of cold and warm periods. Taking into account the above factors, eleven of these warm periods (also called interglacials or interglacials) could be identified and described in detail during the last 800,000 years.
Abrupt climate changes are a special form of climate change. In Earth's history, they have been triggered by eruptions of super-volcanoes, large-scale magma outflows, greatly increased greenhouse gas emissions, rapid changes in ocean currents or short-term feedback processes in the climate system, often in conjunction with biological crises or mass extinctions. Abrupt climate changes can occur regionally (such as the Dansgaard-Oeschger events in the North Atlantic region during the last cold period) or have global impacts, for example as a result of a large impact event.
The term climate oscillation is sometimes used for climate changes that last only a few decades or are cyclical in nature with a variable period, rarely exerting a global influence. Cyclical fluctuations are also referred to as climate fluctuations, and relatively rapid cyclical changes are also referred to as climate oscillations. An epoch of comparatively cool climate is sometimes called climate pessimum in this context, a relatively warm phase climate optimum or warm optimum. Optimum and pessimum are a convention in climate systematics and not a valuation, but can lead to misinterpretation and are therefore replaced by the term climate anomaly in recent literature. The Misox oscillation (international 8.2 kiloyear event) that occurred during the early Holocene in parts of the Northern Hemisphere, probably caused by a massive input of meltwater into the North Atlantic, was a sharply limited in time but relatively pronounced climate anomaly.
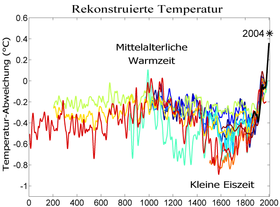

Ten different reconstructions of temperature changes over the last 2000 years (data smoothed, error bars of the individual reconstructions are not shown).
_720p_HD_50FPS.webm.jpg)

Play media file Temperature curve of the Earth over the past 500 million years (simplified reconstruction). For a more detailed presentation including the methods used, see here.
Causes of natural climate change in the Earth system
Climate changes in the history of the Earth have often been based on a combination of several factors. Most of these climate factors are now well understood scientifically and some have been proven by measurement, others are generally accepted as fundamental causal links, and some are obvious due to good correlations of the presumed influencing variables with certain climatic developments, but have not yet been definitively clarified in detail. In general, a distinction is made between positive and negative feedbacks, with positive being self-reinforcing feedbacks (such as ice-albedo feedback or water vapour feedback) and negative being self-attenuating or stabilising feedbacks. A negative feedback system will thus compensate for disturbances in its energetic balance and return to the original climate state.
Even during a geologically uneventful period, the climate was never really stable and, even apart from the major environmental crises, was subject to significant fluctuations over periods of several 10,000 or 100,000 years. The main reasons for this are changes in vegetation cover with repercussions on albedo and carbon cycle, longer-lasting volcanic activities with corresponding release of CO2, aerosols and sulphur dioxide, or regionally occurring plate tectonic processes such as the opening or closing of sea lanes, each associated with a shift, intensification or weakening of atmospheric and oceanic circulation patterns.
Some hypotheses argue that on the scale of the Earth's history, the course of climate is controlled not only by terrestrial factors, but also by varying cosmic ray influences. Thus, according to this assumption, the cold periods of the Phanerozoic are supposed to correlate with regular spiral arm passages of the sun and its heliosphere. However, periodic cosmic influences on biological and climatic development are only weakly documented according to current research and play at best a subordinate role.
Sun
→ Main article: Main sequence star, Faint young sun paradox, and Star structure.
Of those factors that have shaped the earth's climate from the beginning and continue to do so today, the external influence of the sun on the earth's climate system plays the most important role. The solar energy generated and radiated in a thermonuclear fusion process is the basis for the emergence and development of life on Earth. According to the determination made in 2015 by the International Astronomical Union, the average radiation intensity in the form of the solar constant outside the Earth's atmosphere is 1361 W/m². Due to the eccentricity of the Earth's orbit, its intensity varies between 1325 W/m² and 1420 W/m² during the year. However, the insolation at the Earth's surface is much lower and amounts to about 700 W/m² in summer midday sun in Central Europe under clear skies, whereas in winter it is only about 250 W/m².
The term solar constant is somewhat misleading, since it is subject to cyclical fluctuations (about 0.1 percent both in the visible range and in the total radiation), albeit within narrow limits, and is causally linked to the maximum and minimum periods of the sunspots and thus to the different periods of solar activity.
These fluctuations are based on more or less regular changes of the solar magnetic field and are accompanied by a visible fluctuation of the sunspots. The two main cycles are the Schwabe cycle (11 years) and the Hale cycle (22 years). In addition to the Gleissberg cycle (85 ± 15 years), a number of longer-term cycles have been postulated. These are essentially
- the Suess or de Vries cycle (180-210 years),
- a 1470-year cycle that may correlate with the Dansgaard-Oeschger events of the last cold period,
- the Hallstatt or Bray cycle (2400 ± 200 years), possibly the effect of a constellation of the major planets (gas giants) in the solar system occurring every 2318 years.
However, the sun can also record reduced activity for decades and remain in a "standstill phase", so to speak. The English astronomer Edward Maunder examined the historically documented number of sunspots in 1890 and found a pause in the 11-year cycles between 1645 and 1720 (Maunder minimum), which was roughly in the middle of the so-called "Little Ice Age". However, cooler climate periods in historical times (as well as warm periods such as the medieval climate anomaly) were regionally and temporally unevenly distributed and occurred globally only rarely and for only a few decades. Accordingly, the core phase of the Little Ice Age - from the end of the 16th to about the middle of the 19th century - was very probably confined to the northern hemisphere in varying degrees. This puts the influence of the sun into perspective, since factors such as volcanic activity, changes in atmospheric circulation and the North Atlantic Oscillation must be taken into account in addition to fluctuations in solar irradiation.
For more distant epochs, the magnetic activity of the Sun can be determined with the help of the cosmogenic radionuclides 14C and 10Be, which are formed by cosmic radiation. In principle, the C14 method provides more precise results when a calibration curve is used (DeVries effect), but cannot be used for longer time scales due to the comparatively short half-life of the 14C isotope of 5,730 years. In contrast, the half-life of the beryllium isotope 10Be is 1.51 million years and is therefore suitable for an analysis period of up to 10 million years. The concentration of 10Be correlates with cosmic rays and thus indirectly with the strength of the Earth's magnetic field and solar activity. In addition, high 10Be fractions - synonymous with low solar activity - also indicate increased aerosol concentrations in the atmosphere.
The changes in the solar constant and solar activity measured by satellites since 1978 are too small to be considered as an explanation for the temperature development of recent decades. All data sets indicate that since the middle of the 20th century the global temperature development has largely decoupled from solar activity. According to this, the additional radiative forcing by the sun since the beginning of industrialisation amounts to about 0.11 W/m², while anthropogenic greenhouse gases currently contribute about 2.8 W/m² to warming, with an upward trend.
On the entire time scale of Earth and climate history, the evolution of the Sun as a main sequence star has primary importance in the Hertzsprung-Russell diagram. After a relatively short phase as a protostar, it began converting energy 4.6 billion years ago through the process of nuclear fusion, in which the supply of hydrogen present in the solar core is gradually converted into helium by the proton-proton reaction. This stage lasts about 11 billion years, during which time the luminosity and radius of the Sun increase constantly. This means that at the beginning of its existence (and at the same time at the beginning of the Earth's history) the Sun had only 70 percent of its present radiant power, and that this radiant power increases on average every 150 million years by about 1 percent up to the present value and will continue to increase. This so-called Faint Young Sun Paradox not only embodies an elementary climate factor over billions of years, but also leads to fundamental questions about the origin and continuity of terrestrial life, which are currently being discussed on a broad interdisciplinary basis, especially in the atmospheric sciences.
Earth orbit, precession and axial inclination
→ Main article: Milanković cycles
The Earth's orbit around the Sun, the precession of the Earth's axis of rotation, as well as the inclination of the Earth's axis and thus the changing angles of incidence of solar radiation in the northern and southern hemispheres are subject to various cycles with a duration of 25,800 to about 100,000 and 405,000 years, respectively. They were first studied and calculated by the Serbian astrophysicist and mathematician Milutin Milanković (1879-1958) with respect to earth science issues. The fluctuations in insolation on the Earth's surface caused by the Milanković cycles turn out to be relatively minor, but they are responsible for a changing irradiation fraction of the hemispheres with their different land mass fraction and thus act as "pulse generators" in the climate system. They are considered to be the main cause for the change of warm and cold phases within the present ice age. For example, the slight temperature increase initiated by the orbital parameters caused an increase in the atmospheric CO2 concentration, which subsequently led to further warming and to a transition from a cold to a warm period, with both increases being almost synchronous in many cases according to recent studies.
Although the process of gradually changing insolation takes considerable periods of time, it can be demonstrated metrologically over millennia. Sediment cores from the deep sea, for example, provide evidence of a Holocene climate optimum some 8,000 to 6,000 years ago, whose temperature values on a global basis were only reached again in the 21st century and have probably been exceeded in the meantime. Due to the decrease in solar radiation in northern latitudes during the summer maximum, coupled to the periodicity of Milanković cycles, a slight temperature decrease of 0.10 to 0.12 °C per millennium on average has taken place since then. This cooling trend would normally result in a new cold period following the Holocene interglacial in 30,000 to 50,000 years. Whether this event occurs as predicted or whether the current warm period will be of longer duration depends largely on the extent to which anthropogenic and natural greenhouse gases enter the atmosphere in the future.
The periodic changes of the Earth's orbital parameters are detectable as a stable influence over large parts of the Phanerozoic, even in the predominantly tropical climates of the Cretaceous. Thus, according to new analyses, the major cycle of 405,000 years could be traced back to the Upper Triassic, about 215 million years ago, and chronologically classified. Milanković cycles are also thought to have had a significant influence on the climatic fluctuations that occurred during the Permo-Carboniferous glacial period in the Late Carboniferous (about 315 to 299 mya). According to recent findings, the periodic changes in eccentricity could also influence the carbon cycle within the different Earth spheres. This is particularly true for the climate evolution during the Cenozoic, using the eccentricity cycle as a temporal scale for a more detailed analysis of the different climate states and their transitions.
For decades, experts took little notice of Milanković's calculations, which were considered speculative. Since the 1980s, however, the theory has become an integral part of palaeoclimatology and Quaternary research in modified and extended form, and is frequently used as an important factor influencing geological history and as an instrument for reconstructing cold phases. The following table summarizes the most important key data of the Milanković cycles.
Earth orbit parameters | Cycle duration | Fluctuation range | Current status |
precession of the earth's axis of rotation | approx. 025,800 years | 360° (full circle) within a complete cycle | Development towards a more concise formation of the seasons in the northern hemisphere with longer winters |
inclination angle of the earth's axis to the ecliptic | approx. 041,000 years | between 22.1° and 24.5 | 23.43° (with tendency to minimum) |
eccentricity of the earth's orbit | approx. 100,000 or 405,000 years1) | from 0.0006 (almost circular) to 0.058 (slightly elliptical) | 0.016 (with tendency to circular orbit) |
1) Next minimum of eccentricity with 0.0023 in 27,500 years, absolute minimum with 0.0006 in more than 400,000 years
Greenhouse gases
→ Main article: Greenhouse gas
More than 20 greenhouse gases of natural and anthropogenic origin are detectable in the earth's atmosphere, including highly effective climate gases such as nitrous oxide (laughing gas), sulphur hexafluoride and carbonyl sulphide. Although, with regard to concise climate change events of the past, almost exclusively carbon dioxide and methane played a primary role in addition to water vapour, the significance of the other greenhouse gases is certainly relevant, as they currently have almost the same global warming potential as carbon dioxide in their overall impact.
Unlike nitrogen, oxygen and all noble gases, greenhouse gases are active in infrared radiation thanks to their molecular structure. CO2, for example, can absorb solar heat energy at wavelengths of 4.26 µm and 14.99 µm and re-emit it towards the earth's surface. Due to this greenhouse effect, which was first described by Joseph Fourier as early as 1824, the average temperature near the surface increases by approximately 33 °C to +14 to +15 °C in the mathematical-physical model. Without the greenhouse effect, the lower atmosphere would have a global mean temperature of only -18 °C, leading to complete glaciation of the planet (although the temperature level would probably drop even further due to several interactions).
The most important and, in terms of its influence, strongest greenhouse gas is water vapour, whose share in the natural greenhouse effect varies between 36 and 70 per cent depending on geographical conditions or climate zone. Since the atmospheric water vapour content is directly dependent on the air temperature, its concentration decreases at lower average temperatures and increases during a warming phase (water vapour feedback), whereby according to the Clausius-Clapeyron equation the atmosphere can absorb 7 per cent more water vapour per degree increase in temperature.
The atmospheric concentration of carbon dioxide is usually given in ppm (= parts per million), that of methane in ppb (= parts per billion). Due to human influences, since the beginning of the industrial age the level of carbon dioxide has increased to over 400 ppm (previously 280 ppm) and that of methane to almost 1,900 ppb (previously 800 ppb). These are the highest concentrations for at least 800,000 years. In all likelihood, no significantly higher CO2 levels have occurred during the last 14 million years (since the Middle Miocene climate optimum) than in the 21st century to date. Nevertheless, there were geological epochs with significantly higher CO2 levels, such as in the Cambrian period around 500 million years ago, when the carbon dioxide concentration was in the range of 5,000 to 6,000 ppm. Conclusions about the present are problematic, however, because the conditions at that time (including the 4 to 5 percent reduction in solar radiation compared to today, the complete absence of land plants and the associated altered organic carbon cycle) are in no way transferable to the Holocene.
Carbon dioxide and/or methane were not always the main factors in climate change. In Earth's history, they sometimes acted as "feedbacks" that amplified, accelerated or weakened developments that had already begun, depending on the geophysical constellation. In this context, feedbacks such as the ice-albedo feedback, vegetation cover, weathering processes and the variability of the water vapour content in the atmosphere must be considered in addition to the Earth's orbital parameters.
Over the entire duration of the Phanerozoic, the CO2 concentration decreased over the course of 540 million years; it fluctuated strongly in the process. For example, about 300 million years ago during the Permo-Carboniferous Ice Age, at the transition from the Carboniferous to the Permian, CO2 levels averaged 300 ppm, possibly dropping to about 100 ppm in the early Permian. In contrast, 50 million years later, during the super greenhouse phase at the Permian-Triassic boundary, the CO2 equivalent reached a level of about 3,000 ppm in a geologically very short time due to large-scale flood basalt outflows and other feedback processes.
Based on the findings and data of paleoclimatology, the scientific consensus is that the climate change currently being observed is predicted to proceed more rapidly than any known warming phase of the Cenozoic (i.e., during the last 66 million years). Even during the thermal anomaly of the Paleocene/Eocene Temperature Maximum (PETM), atmospheric carbon input and the coupled temperature increase had significantly lower annual average rates of increase than at present.
In contrast to earlier assumptions, the additional anthropogenic CO2 input will only decrease gradually even if emissions are largely halted and will still be detectable to a significant extent in several thousand years' time, since carbon dioxide in the Earth's atmosphere is only degraded very slowly by natural physical and biogeochemical processes in the Earth system. The German Federal Environment Agency assumes that after 1000 years about 15 to 40 percent will still remain in the atmosphere. Based on this factual situation, some studies postulate a longer warm period in the range of 50,000 to 100,000 years, taking into account Earth system climate sensitivity. Various tipping elements in the Earth system have been identified as additional potential hazards that would trigger a series of irreversible processes in the short term if warming continues to increase. A simulation published in 2019 suggests that when CO2 concentrations exceed 1,200 ppm, stratocumulus clouds could break up, contributing to the intensification of global warming. Under these conditions, this process could have come into play during both the strong warming phases of the Eocene and the Upper Cretaceous climate optimum.
Plate Tectonics
→ Main article: Plate tectonics, continental drift and supercontinent
Plate tectonics, as the "driving force" of all large-scale processes in the Earth's outer shell (lithosphere), is one of the most important climate factors on a geological scale, with a large number of associated processes and effects. These include the formation of folded mountains (orogeny), the various forms of volcanism (hotspots or mantle diapirs, large magmatic provinces, etc.), the formation of mid-ocean ridges, the "dipping" of oceanic crust under continental lithospheric plates (subduction), and continental drift, each with direct consequences for the atmospheric concentration of greenhouse gases and thus for the climate state of the Earth.
According to geographical definition, there are seven continents on Earth, whereby their current position and number is the result of a development that began more than 150 million years ago. During the Paleozoic Era and over parts of the Mesozoic Era, however, large and supercontinents shaped the topographical picture of the Earth. A supercontinent is a land mass that unites almost all continental plates. The youngest supercontinent in geological terms, Pangaea, was formed by the fusion of the two large continents Laurussia and Gondwana and existed from the Upper Carboniferous to the Mesozoic (310 to 150 million years ago). The collision of the continental plates led to the folding of the crustal rocks and the formation of a high mountain range along the plate boundaries. As conditions stabilized, weathering and ablation processes became a relevant climate factorː They removed large amounts of carbon dioxide from the atmosphere and in this way tended to contribute to global cooling. Millions of years later, after a phase of tectonic quiescence, the continental shields broke apart again at their "seams" with a considerable increase in flood basalt volcanism, which led to a renewed increase in CO2 concentration.
Characteristic of large and supercontinents are a pronounced continental climate with an annual temperature amplitude of up to 50 °C, extensive arid and desert areas in the interior, and a relatively low diversity of fauna. At the peak of its expansion, Pangaea stretched from the north polar region to Antarctica and, including all shelf seas, had an area of 138 million km², of which 73 million km² were accounted for by the southern continental Gondwana. Gondwana, which dominated the southern hemisphere for a long time, was formed about 600 million years ago and included the core areas (cratons) of South America, Africa, Antarctica, Australia, Arabia, Madagascar, New Guinea and India. During its geological history, large areas of Gondwana were covered by glaciers and ice sheets several times, first during the Ordovician Ice Age (also called the Hirnantian Ice Age or the Andean-Saharan Ice Age). It began about 460 million years ago in the Upper Ordovician, peaked during the last Ordovician stage of the Hirnantian, and ended in the Lower Silurian 430 million years ago.
During the Permo-Carboniferous Ice Age (Karoo Ice Age), Gondwana again became the centre of extensive glaciations. This affected what is now southern Africa and large parts of South America between 359 and 318 million years ago. In a second glaciation phase in the Pennsylvanian between 318 and 299 million years ago, the ice sheets shifted to the cratons of India and Australia, before southern Africa glaciated again during the Dwyka Glaciation (until 280 million years ago). The Permocarbon Ice Age was the second longest ice age in Earth's history. It covered a large part of the Carboniferous and ended during the Permian, about 265 million years ago. The position of Gondwana in the vicinity of Antarctica, which hardly changed over millions of years, contributed significantly to the development of the two Paleozoic glacial periods, since mainland areas near the poles ice up faster and more effectively than open ocean zones due to the relatively high albedo, and this process gains its own momentum through the ice-albedo feedback.
Like almost all natural climate change, the Permo-Carboniferous Ice Age event was based on several factors. In addition to the continental glaciation described above, these were the following mutually reinforcing mechanisms:
- Due to the further increase in vegetation cover during the Carboniferous in combination with the spread of deep-rooted and soil-splitting plants as well as extensive incarbonation processes, the atmospheric CO2 concentration fell to an unprecedented low. This development contributed significantly to the fact that towards the end of the epoch and in the early Permian several pronounced climate changes took place, with a fluctuation range of the CO2 level from 150 to 700 ppm coupled to the different cold and warm phases.
- Due to the extremely high oxygen content of 33 to 35 percent, probably the most devastating forest and wildfires in the history of the earth occurred in the Upper Carboniferous, with the possible side effect of a world-wide smoke and haze that dampened the sunlight. P. 443 f.
- After Laurussia and Gondwana united to form the supercontinent Pangaea and thus a huge continental barrier, the water and heat exchange of the equatorial ocean currents stopped, and Antarctic cold water flowed north along the coasts of Gondwana. This contributed to further intensifying the already prevailing cooling trend.
Another example of the climatic relevance of plate tectonics is provided by recent Earth history with the formation of the Drake Strait, which today is about 480 nautical miles wide and connects the Atlantic Ocean with the Pacific Ocean. Until the late Eocene, a land bridge existed between Antarctica and South America - as an extensive remnant of the former Gondwana continent - which became increasingly fragile due to several plate tectonic processes, before the Drake Strait began to open under constant deepening. This created the strongest ocean current on Earth in the Southern Ocean, the Antarctic Circumpolar Current, which from then on circled Antarctica in a clockwise direction, cutting off the continent from the supply of warmer seawater and creating the basis for the formation of the Antarctic Ice Sheet. Thus Antarctica was not only geographically isolated, but thermally isolated as well. The first significant glaciation at the Eocene-Oligocene boundary 33.9 million years ago was synonymous with the beginning of the Cenozoic Ice Age, and during the Pliocene the ice cover first reached its present extent of about 14 million km².
Volcanism
Volcanic eruptions of magnitude 5 or 6 on the Volcanic Explosivity Index have the potential to cause aerosol-induced global cooling of about -0.3 to -0.5 °C over several years, associated with multiple feedbacks, as was demonstrated for the 1991 eruption of Pinatubo, among others. In particular, gases can reach the stratosphere (17 to 50 km altitude). Through three processes, known as gas-to-particle conversion (GPC), drop-to-particle conversion (DPC) and bulk-to-particle conversion (BPC), ejected particles and gases become aerosols. Due to the high-altitude currents (strong wind bands), these spread into the stratosphere, where they change the transmitted solar radiation via absorption, scattering and reflection. These processes have a direct influence on the temperature in all air layers.
The effects of a volcanic eruption can vary greatly over time. Depending on the formation process, aerosols have typical radii from r < 0.1 μm to r > 1 μm. Depending on the radii and the corresponding cleanup mechanisms, aerosols have residence times that span periods from a few minutes to several years before they are removed from the atmosphere by leaching (ice, snow, or rain), deposition by gravity, or coagulation (coagulation, small particles coalesce to form a large particle). This results in a net time-varying effect on air temperature. First, the large particles absorb solar radiation and thus warm the atmosphere (positive net tea effect), but then quickly fall out of the air column. Then the small and medium sized particles become more important as they reflect and scatter solar radiation, causing the air temperature to drop (negative net effect). This negative net effect is also referred to as volcanic winter when it is more pronounced.
Two volcanic eruptions occurring at close intervals were probably the triggers of the pronounced climatic anomaly of the years 536 to 550, which possibly extended into the 7th century due to various feedbacks, cooled large parts of the earth's surface and led regionally to droughts and crop failures (Late Antique Little Ice Age). The eruption of Laki Crater on Iceland in the summer of 1783 is considered the probable cause of the extremely cold winter of 1783/84 in northern Europe and North America. In April 1815, the eruption of the Tambora volcano on the island of Sumbawa, now part of Indonesia, was a major factor in the "year without a summer" (1816). The cold spell mainly affected large areas of North America as well as western and southern Europe. At present, annual volcanic CO2 emissions range from 180 to 440 megatons. Anthropogenic CO2 emissions are several orders of magnitude higher, reaching around 36 gigatons in each of the last few years.
See also: List of major historical volcanic eruptions
Supervolcanoes
Due to their ejection volume of over 1000 km³ of lava, ash and aerosols (tephra), supervolcanoes influenced the climate for decades in prehistoric times and triggered abrupt global cooling. On the Volcanic Explosivity Index, they are rated VEI-8, the highest category. Unlike most other volcanoes, supervolcanoes do not leave behind volcanic cones after an eruption, due to the size of their magma chamber, but huge calderas. The last eruption of a supervolcano occurred on the northern main island of New Zealand about 26,500 years ago in the area of today's Lake Taupo. Another eruption occurred with the Toba explosion 74,000 years ago on Sumatra. Currently, there are several potential supervolcanoes that could reach VEI-8 category if they erupt again. The best known of these is located beneath Yellowstone National Park in the US state of Wyoming. The history of this hotspot can be traced back over 17 million years and records a series of eruptions during this time, including two supereruptions in the younger Miocene (8.99 and 8.72 mya). Since the beginning of the Oligocene (33.9 mya), more than 40 such events have been clearly recorded worldwide. However, permanent climatic and ecological consequences due to supervolcanoes have not been documented.
Large Magmatic Provinces
In the context of geological history, so-called Large Igneous Provinces were the cause of profound and relatively rapid climate change events. These are large-volume ejections of magmatic rocks from the Earth's mantle, predominantly in the form of flood basalts, which occasionally spread over millions of km² in the course of several hundred thousand years. Depending on the extent and duration of the flood basalt release, considerable amounts of carbon dioxide were released into the atmosphere, as well as significant amounts of hydrogen chloride, fluorine and sulphur dioxide. In contrast to "normal" volcanism, the activities of a major magmatic province did not cause aerosol-induced cooling, but on the contrary led to a global temperature increase, in extreme cases coupled with an additional warming spiral involving methane or methane hydrate from oceanic deposits. It is very likely that most mass extinctions in Earth's history are directly related to the large-scale outflow of flood basalts and the subsequent destabilization of terrestrial and marine biotopes.
Well-known major magmatic provinces that have had varying degrees of influence on climate and biodiversity include the Siberian Trap (Permian-Triassic boundary, 252 mya), the Deccan Trap in what is now the West Indies (Cretaceous-Paleogene boundary, 66 mya), and the North American Columbia Plateau Basalt (Middle Miocene, major activity 16.7 to 15.9 mya).
Other factors affecting the climate
Other factors that can influence the climate in the long term or have done so in the past:
- The albedo as a measure of the reflectivity of non-self-illuminating surfaces, in the Earth system dependent on the extent of the oceans, ice sheets, deserts and vegetation zones.
- Organisms that have caused climate-altering effects over the course of Earth's history by fixing or producing greenhouse gases, such as corals, methane producers, the phytoplankton, and plants such as the floating fern Azolla
- Changes in the atmospheric circulation, monsoon
- Changes in ocean currents: Thermohaline circulation, North Atlantic Oscillation, Southern Oscillation Index, El Niño (ENSO).
- Sea-level fluctuations (eustasia), caused either by the binding of water in continental ice sheets (or their melting) or by changes in ocean basin volume due to tectonic shifts.
- The heat content of the oceans
- The moon through its influence on the tides and thus on the major ocean currents
- The temperature-dependent water vapour content of the atmosphere and cloud formation
- Vegetation cover in its function as a carbon sink
- Weathering processes bind atmospheric CO2 in the lithosphere over longer periods of time (carbonate-silicate cycle) and have different effects depending on the respective environmental conditions such as warm or cold periods.
- The positive feedback process of ice albedo feedback
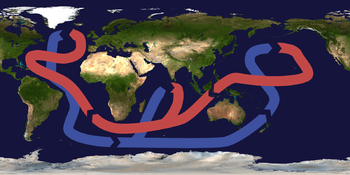

The present Thermohaline Circulation (without Antarctic Circumpolar Current)

The Columbia Plateau Basalt, a major igneous province in the western USA active mainly during the Miocene
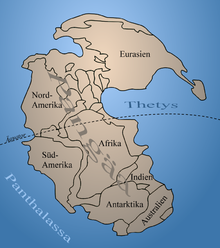

The supercontinent Pangaea in the Lower Permian about 280 million years ago.
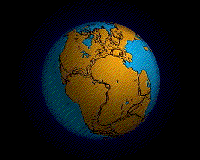

Continental drift of the last 150 million years
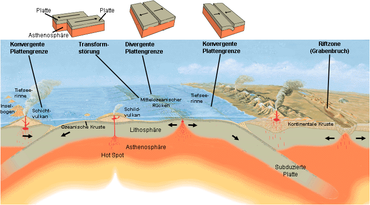

Schematic representation of the processes along the plate boundaries and the associated tectonic activities
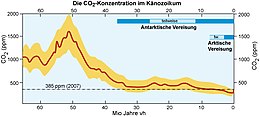

The CO2 content of the atmosphere over the last 60 million years up to the year 2007. The glaciation of the Arctic and Antarctic during the Cenozoic Ice Age falls within this period.
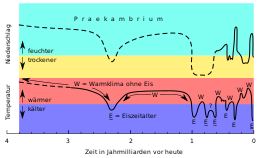

Climate changes in the course of climate history
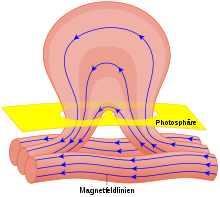

Formation of a sunspot: Bundled magnetic field lines penetrate from the interior of the sun to the surface.
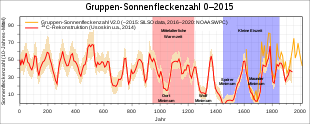

Reconstructed solar activity of the last 2000 years
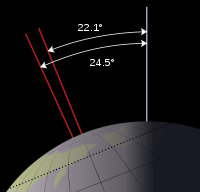

Maximum and minimum inclination range of the earth axis
Anthropogenic climate change
→ Main article: Global warming and consequences of global warming
In addition to natural factors, humans have had a significant and increasing influence on the climate, especially since the beginning of industrialization: The Intergovernmental Panel on Climate Change (IPCC), which summarizes the state of the science on behalf of the United Nations, concluded in 2007 that the warming of the atmosphere and oceans since the beginning of industrialization is primarily due to the release of greenhouse gases by humans, with increasing carbon dioxide concentrations and their measurable influence on the radiation balance being the main factor in the warming process. Recent analyses conclude that anthropogenic climate gas emissions in the 21st century to date exceed those of the Paleocene/Eocene temperature maximum by an annual average of about ten times. By the end of the 21st century, depending on various factors such as the further development of emissions, the IPCC expects a temperature increase in the likely range of 2.6°C to 4.8°C (mean=3.7°C) in the worst case scenario (representative concentration pathway RCP 8.5), which relies very heavily on the use of fossil fuels. In the most favourable scenario (RCP 2.6), which models very ambitious climate protection measures, the likely range is 0.3 °C to 1.7 °C (mean=1.0 °C).
The IPCC writes in its 2014/2015 Fifth Assessment Report that it is extremely likely that humans caused more than 50 percent of the warming observed 1951-2010. The best estimate is that the human influence on warming is roughly consistent with the total observed warming during that period. A 2014 analysis puts the probability that the increase in global temperature recorded over the past 60 years would have been similar in the absence of anthropogenic greenhouse gas emissions at just 0.001%. Several studies agree that, in contrast to pre-industrial climate fluctuations, the current warming process is occurring simultaneously on all continents, is unsurpassed in its rapid development by any climate change in the last two thousand years, and is probably without comparable example in recent Earth history. A detailed evaluation of paleoclimatological data series yielded the result that the warming that has taken place in the 21st century so far exceeds the temperature values of the Holocene climate optimum (about 8000 to 6000 years ago) with a high degree of probability.
Climate change, which is expected to intensify further in the coming decades, has the potential to trigger not only serious environmental changes but also global conflicts and migration movements on a considerable scale ("climate" or "environmental refugees"). A key aspect of current developments is climate protection as the overarching term for those measures that are intended to mitigate and, if possible, prevent the foreseeable consequences of global warming. The primary task here is the sustainable and rapid reduction of anthropogenic CO2 emissions.
World Meteorological Organization (WMO) Secretary-General Petteri Taalas stated in late 2018 that "we are the first generation to fully understand climate change and the last generation to be able to do something about it."
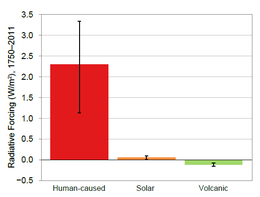

Causes of currently observed global warming (period 1750-2011) (as of 2018).
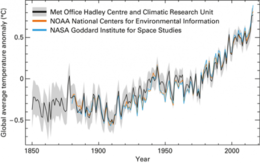

Global average temperature anomaly 1850-2016
Questions and Answers
Q: What is climate change?
A: Climate change is the climate of Earth changing, with temperatures becoming hotter and colder than they are today.
Q: What causes climate change?
A: Climate change this century and last century is sometimes called global warming, because the average temperature on the surface has risen. This is because people are putting heat-trapping greenhouse gases in Earth's atmosphere.
Q: How long has climate been changing for?
A: The climate of the Earth has changed over not just thousands of years, but tens or hundreds of millions of years.
Q: When was it much hotter than it is today?
A: About 60 million years ago there were a lot of volcanoes which burnt a lot of underground organic matter, making the Earth hot enough for giant tortoises and alligators to live in the Arctic.
Q: When was it much cooler than it is today?
A: At times in the past, the temperature was much cooler, with the last glaciation ending about ten thousand years ago. Ice Ages are long times when the Earth got colder, and more ice froze at the North and South Poles. Sometimes even the whole Earth was covered in ice, and was much colder than today.
Q: What causes Ice Ages?
A: Changes in the Earth's orbit around the Sun, how much sunlight reaches us from different angles due to how tilted our planet is compared to its orbit around sun as well as activities from living things can cause Ice Ages.
Q: What caused global warming before humans existed? A: Before people existed, carbon dioxide and methane went up into earth's atmosphere due to burning underground organic matter like coal gas oil which made earth hot enough for giant tortoises and alligators to live in arctic regions thus causing global warming without human intervention