Chromosome
Chromosomes (from ancient Greek χρώμα chrōma 'colour' and σώμα sōma 'body') are components of complex ('eukaryotic') cells on which the hereditary information necessary for the inheritance of characteristics is stored. A chromosome contains deoxyribonucleic acid (DNA), on which genes are encoded, and various proteins (proteins), especially histones. In eukaryotic organisms, which include plants, fungi, animals and thus also humans, chromosomes are present as regular components in the cell nucleus.
The name chromosome, literally "coloured body", comes from the fact that these structures, consisting of chromatin, can be easily stained with basic dyes.
Without special detection methods, chromosomes can only be seen under a light microscope during the division of the cell nucleus. Then they have a rod-shaped appearance in humans and many other species. This condensed form is formed at the beginning of nuclear division in mitosis and meiosis during the early prophase. In the subsequent metaphase, the highly condensed chromosomes are arranged equatorially. In this phase, each chromosome consists of two identical chromatids, each of which contains a continuous DNA double helix, created by replication and arranged parallel to each other. In the later nuclear division phases, the two chromatids of a chromosome are separated from each other and finally assigned to the forming daughter nuclei.
At the end of the nuclear divisions, the chromosomes again enter a decondensed state. Only in this form can the DNA now be read or then duplicated. However, the different chromosomes of a nucleus can no longer be distinguished using classical staining methods and form an apparently continuous chromatin. With special methods, however, such as fluorescence in situ hybridization, the chromosomes can still be detected as separate units. Thus, each of the decondensed chromosomes occupies a definable region within the nucleus during interphase between nuclear divisions, a chromosome territory. In addition to chromosomes in the nucleus, DNA is also found in some organelles of the cell such as mitochondria in eukaryotes, and in chloroplasts in plants and algae.
Prokaryotic organisms, i.e. bacteria and archaea, do not have a nucleus and do not have chromosomes in the classical sense. Here, the carriers of hereditary information are one or more mostly circular DNA molecules, which are sometimes referred to as bacterial chromosomes. In the mitochondria and chloroplasts of eukaryotes, the DNA is also usually circular and resembles a bacterial chromosome (cf. endosymbiont theory). Their DNA (called chondriome and plastome, respectively) is sometimes formally listed as an additional, non-nuclear chromosome. The packaging of the long DNA molecules in a very small space is similar (homologous) to the nucleus of eukaryotes in archaea, but similar to the organelles of bacteria (see endosymbiont theory).
In the case of viruses whose genome consists of one or more nucleic acid molecules (DNA or RNA), these segments are also sometimes referred to as chromosomes. For example, the RNA genome of influenza A viruses consists of eight such segments (chromosomes).
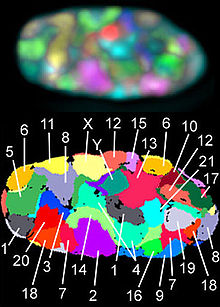

Top: Nucleus of a human fibroblast in which all 23 different chromosomes (1-22, X/Y) were stained by fluorescence in situ hybridization (FISH) with a different combination of a total of 7 fluorochromes. Shown is a middle plane in a deconvoluted image stack acquired by fluorescence microscopy. Bottom: False-color representation of all chromosome territories visible in this focal plane, according to computer classification.
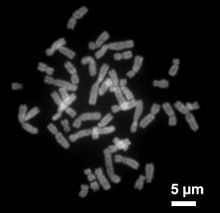

Metaphase chromosomes from a human female lymphocyte cell, stained with the fluorescent dye chromomycin A3. The chromosomes are partially superimposed. Each metaphase chromosome is composed of two daughter chromatids, which are separated longitudinally by a dark gap.
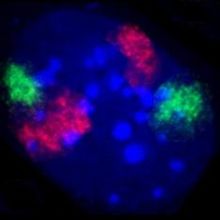

Nucleus of a mouse fibroblast. The territories of chromosomes 2 (red) and 9 (green) were stained by fluorescence in situ hybridization. DNA counterstaining in blue.
Research History
The name chromosome was proposed in 1888 by the anatomist Heinrich Wilhelm Waldeyer, after Walther Flemming had introduced the term chromatin for the colourable substance in the cell nucleus a few years earlier. As late as 1906, Oscar Hertwig used the term nuclear segments in parallel, which was intended to clarify that during the division of the nucleus (mitosis) "the chromatin is broken down into segments". Another old term, which was also used in parallel with chromosome for a while, is nuclear loop, for example by Karl Heider (1906).
The story of the discovery of chromosomes and their function cannot be separated from the previous discovery of the nucleus.
In 1842, the Swiss botanist Carl Wilhelm von Nägeli described "transitory cytoblasts" (stainable rod-shaped structures in the nucleus of plant cells), which were probably chromosomes. Illustrations from the works of other researchers can also be interpreted with today's knowledge as chromosomes or mitotic cell division (Matthias Schleiden 1846, Rudolf Virchow 1857, Otto Bütschli 1873).
In 1873 Anton Schneider described on flatworms that the cell nucleus changes "into a heap of finely curved threads which become visible on the addition of acetic acid. In place of these thin threads, thick strands finally appeared, at first irregular, then arranged into a rosette, which lies in a plane passing through the center of the sphere (equatorial plane)." "Indirect nuclear division" (mitosis) had been discovered - but not yet understood. In 1882, for example, Walther Flemming still assumed that the "nuclear filaments" separated from each other only during the early phase of nuclear division from a previously continuous filament. Although he observed a longitudinal splitting of chromosomes at a later stage (today called metaphase), he assumed that whole chromosomes (i.e. with both chromatids) move towards the future nuclei later (today: anaphase). Also, he did not exclude that cell nuclei could also be newly formed, at least in some cases, i.e. not by division from existing nuclei. In 1884, several authors (L. Guignard, Emil Heuser and Edouard van Beneden) described the division of the chromosome halves (today: chromatids) into daughter cell nuclei.
Since chromosomes were not visible during interphase, it was initially unclear whether they dissolved after a nuclear division and reformed before each nuclear division or whether they persisted in the nucleus as separate units in each case. The latter idea was called the doctrine of the maintenance of individuality of chromosomes and was proposed by Carl Rabl (1885). He was also the first to establish, first, a constant number of chromosomes in different mitoses of a tissue and, second, to conclude from this that the chromosomes must also be present in interphase and thus continuously. However, he initially left open the possibility that this number could be different in different tissues. Rabl was also the first to assume that each chromosome in the interphase nucleus forms its own territory.
The idea of chromosome continuity was by no means met with undivided approval. An important opponent was Oscar Hertwig (1890, 1917). Theodor Boveri, on the other hand, endorsed Rabl's ideas and supported them with further experimental findings (1904, 1909). Also in the 1880s, August Weismann developed his germplasm theory (see also there), in which he assumed that the hereditary material was localized (only) in the chromosomes. Important conclusions were that inheritance took place exclusively via the germ line and that inheritance of acquired characteristics should be rejected. What later turned out to be largely correct was hotly disputed at the time. An unsparing critique can be found, for example, in Meyer's Konversations-Lexikon of 1888 under the keyword heredity.
In 1900, Mendel's rules were rediscovered and confirmed. Subsequently, the new science of genetics developed, in the context of which the connection between chromosomes and heredity was demonstrated many times. For example, in 1910 Thomas Hunt Morgan was able to demonstrate on Drosophila melanogaster that chromosomes are the carriers of genes. In 1944, Oswald Avery (see there) showed that the actual hereditary molecule is DNA, and not proteins in the chromosomes.
The further history until 1950 (elucidation of the structure of DNA) is described in the article Chromosome theory of heredity. A timeline of some important discoveries can be found in the article Chromatin.
In 2000, two international teams of scientists deciphered most of the human genome; by 2003, 99 percent had been sequenced. With chromosome 1, the last of the 24 different human chromosomes was precisely analysed in 2005/2006 (99.99%). More than 160 scientists from Great Britain and the USA published this collaborative work.
In 2014, the design and construction of a synthetic chromosome was achieved for the first time, in the baker's yeast Saccharomyces cerevisiae.
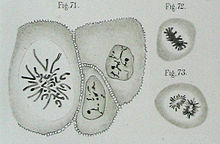

Cell division stages in the cornea of the eye. Probably the oldest representation of human chromosomes. Walther Flemming, 1882.
Chromosome assembly and structure
Components
Apart from special cases (see giant chromosomes below), a chromosome in the simple case contains a continuous DNA double strand (also: DNA double helix). The DNA double strand is sometimes referred to as a DNA molecule, although strictly speaking it is two single stranded molecules (see deoxyribonucleic acid). Histones and other proteins attach to the DNA double strand (see below). The mixture of DNA, histones, and other proteins is called chromatin. A chromatid is built from a DNA double strand by this protein attachment. In this case, the chromosome therefore consists of a chromatid. The described case always occurs directly after a nuclear division; in most animals and plants additionally in all cells that can no longer divide (exception: polytic chromosomes in insects, see also below), and in cells that temporarily stop growing, i.e. are in the G0 phase (see cell cycle).
When a cell grows in order to divide later, the DNA must be duplicated ("replicated") in a certain section of the cell cycle (S phase). This is necessary so that both daughter nuclei can later receive the entire genetic material, i.e. one copy each of all chromosomes. After DNA duplication, each chromosome has two identical DNA double strands. These two double strands are spatially separated from each other and packaged with proteins: two sister chromatids are formed. During nuclear division (mitosis), the two sister chromatids of a chromosome become microscopically visible as units that run parallel but are separated by a narrow gap (see schematic drawing on the right and first figure of the article). At a point called the centromere or centromere, each chromosome is narrower at this point than in the rest of its course: Here, the sister chromatids still hang together. As mitosis progresses (at the transition from metaphase to anaphase, see below), the two sister chromatids are separated - with two daughter chromosomes resulting from the separation - and distributed to the newly forming nuclei: The chromosomes in these new nuclei now consist of one chromatid again. Accordingly, a chromatid always contains exactly one DNA double strand, whereas a chromosome contains one or two DNA double strands, depending on the phase of the cell cycle, and accordingly consists of one or two chromatids (exception: the polytene chromosomes mentioned, which can contain over a thousand double strands).
The centromere divides the chromatids into two arms. Depending on the position of the centromere, we speak of metacentric chromosomes (centromere in the middle), acrocentric chromosomes (centromere at the end, the shorter arm very small; in humans chromosomes 13, 14, 15, 21, 22 and the Y chromosome) or submetacentric chromosomes (centromere between middle and end). The shorter arm is called the p-arm (French petit 'small'), and the longer arm is called the q-arm (q follows p in the Latin alphabet). As in the schematic drawing, chromosomes are generally shown with the short arms pointing upwards.
The ends of the chromosomes are called telomeres (singular: telomere). They contain a short, identically repeating DNA sequence (in humans TTAGGG). There chromosomes become a little shorter with each doubling. Telomeres therefore play an important role in ageing processes. Besides centromere and telomeres, starting points for DNA duplication (replication) are the third essential component of a chromosome (see ARS element).
In humans, the short arms of acrocentric chromosomes contain genes for ribosomal RNA. These short arms may be extended by a satellite in condensed metaphase chromosomes, so that satellite chromosomes (SAT chromosomes) are present (not to be confused with satellite DNA). The genes for ribosomal RNA are present in many copies in tandem. In the interphase cell nucleus, the nucleolus forms on these. Therefore, they are also called nucleolus organizing regions (NOR).
Chromosomes during normal nuclear division (mitosis)
→ Main article: Mitosis
The following is a brief description of the stages during mitosis:
- Prophase: In this first stage of mitosis, chromosomes increasingly condense. They are thus transformed from an accessible source of genetic information to a compact transport form that can no longer be read. The nuclear membrane is dissolved. This is sometimes seen as the beginning of an additional phase, the prometaphase.
- Metaphase: The chromosomes migrate to the equatorial plane of the cell and form the metaphase plate there. Up to this point, each chromosome consists of two chromatids. Centrioles are located at the opposite poles of the cell, the plasma fibers have formed the spindle apparatus.
- Anaphase: The spindle apparatus ensures the separation of the two chromatids of each chromosome and their transport vertically away from the metaphase plate, to two opposite cell poles. For this purpose, microtubules are attached to the kinetochores of the centromeres as well as to the cell poles, acting like stretch ropes.
- Telophase: After completion of the anaphase movement, a new nuclear envelope is formed around each separated chromosome and decondensation begins. Fusion of the particles gives rise to the two daughter cell nuclei, which now contain single-chromatid chromosomes.
After nuclear division, cell division, cytokinesis or cytokinesis, usually also occurs, but this is no longer counted as mitosis.
G-, R- and other chromosome bands
| Human chromosome 1 from a metaphase preparation with G-banding. The end of the p-arm (top) is very bright, it consists of gene-rich R-bands. Compare also chromosome 1 in the next figure. The gap between the sister chromatids is not visible in this example. |
In the mid-20th century, techniques were developed to "spread" chromosomes from cells that were in metaphase: In the resulting metaphase preparation, the chromosomes of a cell lie side by side on a slide so that they can be counted and compared under the microscope (see first figure above). In well-done preparations, the individual chromosomes have the frequently depicted X-like shape. With classical staining methods such as Giemsa staining, chromosomes are uniformly stained along their entire length. Therefore, it was initially impossible or difficult to reliably distinguish chromosomes of similar size from each other. Around 1970, it was discovered that some areas of chromosomes no longer accept the Giemsa stain if the chromosomes had previously been treated with trypsin. The G banding that was induced created alternating stained sections (the G bands, G for Giemsa) and unstained sections (the R bands, R for reverse) along the chromosomes. The banding pattern makes it possible to unambiguously identify all chromosomes in humans and a number of animals. The material basis for the different staining behaviour of the bands, i.e. the question why some areas do not absorb the dye after trypsin treatment, is still unclear. However, it turned out that G- and R-bands differ in some properties.
R bands contain an above-average number of genes, an above-average number of G-C base pairings and are duplicated early during chromosome replication. In humans, they are rich in Alu sequences (see there and figure on the right).
G bands are gene-poor, the number of G-C base pairs is below average (but they have more A-T pairs; see deoxyribonucleic acid), and they tend to replicate late during chromosome duplication. In humans, they are rich in L1 elements (see Long interspersed nuclear element).
C-bands (the centromere regions) and T-bands are sometimes distinguished as further band types. The latter are a subgroup of the R-bands, are particularly gene-rich and are often located near the telomeres, hence the name.
The number of R and G bands depends on the degree of condensation of the chromosomes. In metaphase, all human chromosomes together have about 400 of these bands, while in the prophase chromosomes, which are not yet so highly condensed, up to 850 bands can be distinguished.
Nomenclature: In order to enable an accurate designation of all chromosomal regions, standardized designation systems have been introduced for humans and some other organisms. In humans, each band has a designation composed of the following elements: Chromosome number, p or q for the respective arm (p as in French petite for the short arm; q as in French queue for the long), and numbers counting upward from the centromere. For finer distinction, the numbers may have multiple digits. Thus, band 3q26.31 is a subband of 3q26. The designation "3q" accordingly stands for the entire long arm of chromosome 3. Centromere regions are also designated by c (3c). For simplicity, telomeric regions are often referred to as tel (about 3ptel or 3qtel) and telomeric regions as ter (3pter). Schematic representations of the standard bands are called idiograms. Examples can be seen in the figure to the right and on the Ensembl website. In idiograms, G-bands are always shown in dark, R-bands in white. Areas of repetitive elements are sometimes shown shaded. A sorted arrangement of all mitotic chromosomes from a cell is called a karyogram (figure below). The karyotype of a living being indicates how many and, if any, which chromosomes that individual has. The karyotype of a female is given as 46,XX, that of a male as 46,XY (see below, sex determination).
Size and gene density
The human genome, i.e. the total length of the DNA, comprises about 3.2 Gbp (= gigabase pairs or billions of base pairs) with 23,700 genes found so far. Humans have two copies of the genome (2n), one from the mother and one from the father, which are present in each cell nucleus. From the molecular model of DNA, the length of 10 base pairs in the double helix is 3.4 nanometers (billionths of a meter). From this, it can be extrapolated that the total length of DNA in each human cell is over 2 meters. In humans, these are distributed over 2n = 46 chromosomes, so that a chromosome contains on average about 140 Mbp (= mega base pairs or million base pairs) and thus a DNA thread of just under 5 cm in length with just over 1000 genes. However, chromosomes during nuclear division have a length of only a few micrometers (millionths of a meter). They are thus shortened or "condensed" by a factor of about 10000. Even in the interphase nucleus chromosomes are hardly longer. The chromosome territories present here arise essentially by decondensation of the daughter chromatids into width. While a daughter chromatid in metaphase has a diameter of about 0.6 micrometers, a chromosome territory may occupy a circumference approximately equal to its length. However, chromosome territories can have very irregular shapes. From the numerical values given, it is clear that chromosomes must also be strongly compacted, i.e. unfolded, during interphase (see next section).
Chromosome 1 as the largest human chromosome has 249 Mbp, the shortest chromosome 21 has less than a fifth of that, 47 Mbp. The genes are unevenly distributed between the chromosomes. The relatively gene-richest chromosome 19 contains about 1500 coding genes on 59 Mbp, while the gene-poor chromosome 18 contains only about 640 on 80 Mbp (see also figure "Gene-rich and gene-poor regions" above). However, the Y chromosome is the most gene-poor, containing only 72 coding genes on 57 Mbp. (Status of the information on sizes and gene densities in this paragraph: December 2015)
In the house mouse (Mus musculus), the differences between the chromosomes are smaller. The 3.5 Gbp genome with 22,600 described genes is distributed over 20 different chromosomes (2n=40) with 61 Mbp (chromosome 19) to 195 Mbp (chromosome 1).
The length of individual chromosomes in other mammals varies greatly, depending on the number. Some have few, large chromosomes (e.g., the Indian muntjac, Muntjac muntjacus: 2n=6 in the female and 2n=7 in the male; thus, the X chromosome here corresponds to two Y chromosomes), others have many small ones (e.g., rhinoceros, Diceros bicornis: 2n=84). However, the exact lengths (in base pairs) are only known for a small number of animals.
Chromosomes of extremely different sizes occur in lizards and birds (see figure). The macrochromosomes are similar in size to mammalian chromosomes. For example, chromosome 1 of the chicken (Gallus gallus) contains 188 Mbp. However, there are also many microchromosomes whose size can be less than 1 Mbp. The transition from macro- to microchromosomes is often fluid, so that the demarcation of the two groups from each other is sometimes made differently. In the chicken, for example, macrochromosomes may comprise chromosomes 1-8 or 1-10. For a pictorial comparison of sizes, see Ensembl. The sizes in Mbp are also taken from there. The terms macro- and microchromosomes were introduced by Theophilus S. Painter in 1921, who studied spermatogenesis in lizards.
Molecular structure and hierarchy of packaging levels
The previous section argues that DNA must be highly coiled or "condensed" during both nuclear division and interphase. However, it is still largely unclear how this packaging is organized. Basic structural proteins, histones, play an important role. DNA, histones and other proteins each make up about one-third of the chromosomal mass. This is also known as chromatin. The use of the term chromatin is particularly common for descriptions of the cell nucleus in interphase, since here individual chromosomes cannot be distinguished from each other without special staining (fluorescence in situ hybridization).
On the lowest packaging level, the DNA thread is wound up in nucleosomes, which contain eight histone molecules (see Fig., sub-figure 2). Nucleosomes have a diameter of about 10 nanometers (nm), hence the term 10-nm fiber. Its structure is often compared to a string of pearls, but with the thread wound around the pearls. In a nucleosome, 146 base pairs of DNA are wound up, plus linker DNA between the nucleosomes. The 10-nm fiber can be detected under the electron microscope, as can the next higher level of packaging, the 30-nm fiber. However, the internal structure of the 30-nm fiber, i.e., how it is assembled by unfolding from the 10-nm fiber, is unclear, as are all higher packaging levels. For the latter, different models are discussed. In the loop model, the 30-nm fiber is assumed to run in large loops attached to a kind of backbone. In the chromonema model, on the other hand, the 30-nm fiber is assumed to thicken by further unfolding, creating sections 120 nm and thicker. How the structural change from the interphase state to the prophase chromosome proceeds is also unclear. In the transition of prophase chromosomes to the even more condensed metaphase chromosomes, there seems to be agreement that this is a spiral unwinding.
The condensation of chromosomes or chromatin is not uniform within the nucleus. Some areas of the nucleus are particularly strongly stained by DNA dyes. Condensation is therefore particularly strong here. These areas are called heterochromatin, whereas less strongly stained areas are called euchromatin. In the more condensed areas, gene activity is hindered or even blocked, see epigenetics.
Giant chromosomes
There are two known types of giant chromosomes, polytic chromosomes and lampbrush chromosomes.
Polytene chromosomes
→ Main article: Polytene chromosome
A special feature with regard to the internal chromosomal structure are the polytic chromosomes. They are known from various insects and have been particularly well studied in the fruit fly Drosophila melanogaster and in Chironomus mosquitoes. They are formed by several rounds of duplication of DNA without subsequent nuclear division (endoreduplication). In contrast to "normal" polyploidy, in polytic chromosomes the multiply replicated DNA strands from both homologous chromosomes (i.e. the copy inherited from the father and the copy inherited from the mother) are arranged in parallel, similar to a cable harness. All copies of a gene are therefore located next to each other.
Lampbrush chromosomes
→ Main article: Lampbrush chromosome
Another form of very large chromosomes occurs in the oocytes of amphibians. Because they resemble a bottle brush or lamp brush in microscopic appearance, they have been named lamp brush chromosomes.
| Polytene chromosomes in a salivary gland cell of Chironomus. Walther Flemming, 1882. | | "Chromatic filament, which is comparable to a bottle brush" (according to today's terminology, a lampbrush chromosome) from the nucleus of an egg cell of the aquatic salamander (Triton). (Entire plate.) Oscar Hertwig, 1906. |
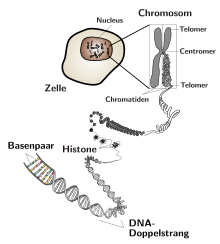

Typical textbook image of chromosomal structure with typical errors. See detailed legend here.
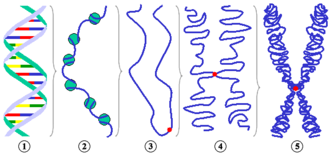

Different levels of chromosome condensation. 1 DNA double helix 2 10-nm-fiber (DNA with nucleosomes ) 3 Schematic of chromatin strand during interphase before DNA duplication with centromere. 4 Condensed chromatin during prophase (now consisting of two chromatids because DNA has doubled). 5 Metaphase chromosomePartial figures 3 to 5 are purely schematic to reflect the number of chromatids during different phases of the cell cycle. The arrangement of the "chromatin thread" does not reflect the actual structure.
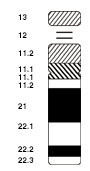

Idiogram of chromosome 21
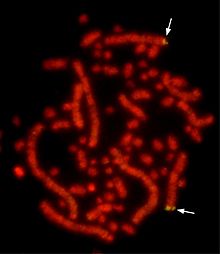

Metaphase chromosomes of the chicken. Typically, birds have microchromosomes that are much smaller than macrochromosomes. Here, fluorescence in situ hybridization was performed to detect a gene locus (β-defensin gene cluster) on the q arm of chromosome 3 (green, arrows). The DNA was stained with the nucleic acid dye propidium iodide (red).
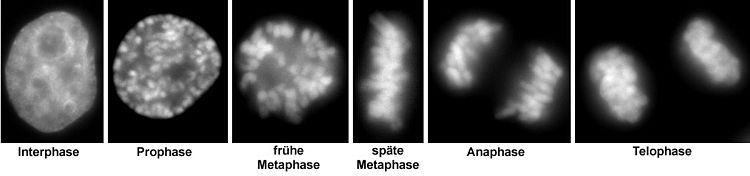

In addition to a nucleus in interphase shown for comparison, the different stages of mitosis are shown.
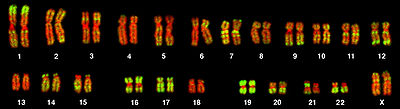

Gene-rich and gene-poor regions on human chromosomes. On metaphase chromosomes from a human female lymphocyte, Alu sequences were marked by fluorescence in situ hybridization (green). These sequences are particularly abundant in gene-rich sections of chromosomes. DNA is stained red, so that gene-poor regions are also visible.
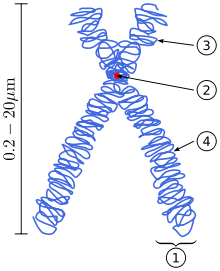

Schematic of a submetacentric metaphase chromosome. 1 One of the two chromatids. 2 Centromere , the site where the two chromatids are joined. This is where the microtubules attach in mitosis. 3 Short arm (p-arm) 4 Long arm (q-arm)
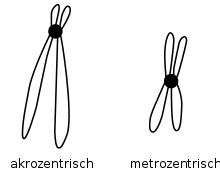

Scheme of an acrocentric and a metacentric metaphase chromosome
Questions and Answers
Q: What are chromosomes made of?
A: Chromosomes are made up of DNA and protein combined as chromatin.
Q: How many chromosomes do humans have?
A: Humans have 46 chromosomes in total, which is 23 pairs.
Q: What determines the sex of a child?
A: The mother's eggs always contain an X chromosome, while the father's sperm contains either a Y chromosome or an X chromosome. That determines the sex of the child. If the child receives a Y chromosome from the father it's male, if it receives an X from the father it is female.
Q: What process reduces diploid cells to haploid cells?
A: To produce sex cells (gametes), stem cells go through a different division process called meiosis which reduces diploid cells to haploid cells.
Q: Where are chromosomes located in a cell?
A: Chromosomes are located in the cell nucleus.
Q: When does mitosis occur?
A: Mitosis occurs when somatic (body) cells divide, such as muscle cells. Before mitosis, the cell copies all its chromosomes and then can divide them into two halves called chromatids which are joined at their centromeres.
Q: Are there any exceptions to having chromosomes in every cell nucleus?
A: There are very few special exceptions to having chromosomes present in every cell nucleus with eukaryotes since only eukaryotes have cell nuclei.