Black hole
This article is about the astronomical object. For the science fiction film, see The Black Hole.
A black hole is an object whose mass is concentrated into an extremely small volume and, as a result of this compactness, generates such strong gravity in its immediate vicinity that not even light can leave or pass through this region. The outer boundary of this region is called the event horizon. Nothing can cross an event horizon from the inside out - no information, no radiation, and certainly no matter. The fact that a "way out" is no longer even conceivable is described conclusively by the general theory of relativity through an extreme curvature of space-time.
There are different classes of black holes with their respective formation mechanisms. The easiest to understand are stellar black holes, which form when a star of a certain size has used up all its "fuel" and collapses. While the outer shells are then ejected in a supernova, the core collapses to an extremely compact body due to its gravitational pressure. For a hypothetical black hole of the mass of the Sun, the event horizon would have a diameter of only about six kilometers, or 230,000ths of the present diameter of the Sun. At the other end of the spectrum, there are supermassive black holes of millions to billions of times the mass of the Sun, which are at the centre of galaxies and play an important role in their evolution.
Outside the event horizon, a black hole behaves like a normal mass body and can be orbited by other celestial bodies on stable orbits. From the outside, the event horizon appears visually as a completely black and opaque object, in the vicinity of which the space behind it is depicted as if distorted by an optical lens.
The term black hole was coined in 1967 by John Archibald Wheeler. At that time, the existence of black holes, which had only been described theoretically, was considered very probable, but had not yet been confirmed by observations. Later, numerous examples of black hole effects were observed, such as the infrared studies of the supermassive black hole Sagittarius A* in the center of the Milky Way beginning in 1992. In 2016, the merger of two black holes via the gravitational waves generated in the process was observed by LIGO, and in 2019, a radio telescopic image of the supermassive black hole M87* at the centre of the galaxy M87 was obtained.
In 2020, scientists Roger Penrose, Reinhard Genzel and Andrea Ghez were awarded the Nobel Prize in Physics for their research on black holes.
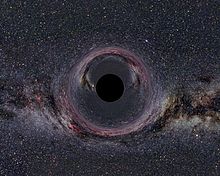

Simulation of a non-rotating black hole of 10 solar masses, as it would look from a distance of 600 km. The Milky Way in the background appears distorted and doubled by the gravitation of the black hole. The image width corresponds to a viewing angle range of about 90°.
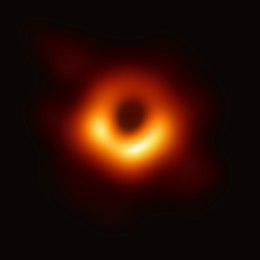

Image calculated from radio images of the Event Horizon Telescope showing the supermassive black hole of the galaxy M87. The black disk in the center of the image is about 2.5 times the size of the event horizon (Schwarzschild diameter about 38-1012 m) of the supermassive black hole in the center.
Research History
→ Main article: History of black holes
18th century
As early as 1783, British naturalist John Michell speculated about dark stars whose gravity was sufficient to trap light. In a letter published by the Royal Society, he wrote:
"If the semi-diameter of a sphere of the same density as the Sun were to exceed that of the Sun in the proportion of 500 to 1, a body falling from an infinite height towards it would have acquired at its surface greater velocity than that of light, and consequently supposing light to be attracted by the same force in proportion to its vis inertiae [mass], with other bodies, all light emitted from such a body would be made to return towards it by its own proper gravity. This assumes that light is influenced by gravity in the same way as massive objects."
"If the radius of a sphere of the same density as the sun exceeded that of the sun in a ratio of 500 to 1, a body falling towards it from an infinite height would have acquired at its surface a velocity greater than that of the light. Consequently - assuming that light is attracted by the same force in proportion to its mass as other bodies - all light emitted by such a body would return to it in consequence of its own gravitation. This is true on the assumption that light is affected by gravity in the same way as massive objects."
- John Michell
The idea of heavy stars, from which corpuscular light could not escape, was also described in 1796 by Pierre Simon Laplace in his Exposition du Système du Monde. He created the term "dark body" (corps obscur) for it. These ideas were within Newtonian physics.
First half of the 20th century: contribution of the general theory of relativity
After Albert Einstein established the field equations of general relativity in 1915, the German astronomer Karl Schwarzschild first gave a metric in 1916, the Schwarzschild metric, which corresponds to the gravitational field of a point-like mass. The Schwarzschild solution describes the size and behavior of a non-rotating and non-electrically charged static black hole with the so-called event horizon at and a central singularity at
. Here
stands for the gravitational constant,
for the mass of the black hole and
for the speed of light.
If, for example, the mass of the sun were compressed into a sphere with a radius of only three kilometres, then no ray of light could reach the outside from its surface. The mass of our Earth ( ) would form a black hole only at a radius of less than one centimeter.
With the Kruskal-Szekeres coordinates in the 1950s, it could be shown mathematically that an external observer who sees an internal observer rushing towards the black hole must get the impression that the internal observer approaches the event horizon only asymptotically, with signals arriving ever more slowly despite regular emission. In contrast, the internal observer itself crosses the event horizon quickly without feeling anything special, although from now on it cannot turn back and its signals cannot reach the external observer. The internal observer is also very soon engulfed by the singularity at .
In the late 1920s, the Indian astrophysicist Subrahmanyan Chandrasekhar showed that a certain limiting mass, called the Chandrasekhar limit, exists for an astrophysical object without nuclear reactions. Objects above this mass limit collapse into neutron stars or black holes, but not white dwarfs as expected. Chandrasekhar's work led to a controversy with astronomer Arthur Eddington. The former believed that stars above the mass limit could collapse into objects whose gravity could trap electromagnetic radiation. Eddington expected, however, that there would be a mechanism to prevent collapse. In 1939, Robert Oppenheimer, together with Robert Serber and George Michael Volkoff, used model calculations to demonstrate that the collapse of a large star would produce a black hole.
Second half of the 20th century: Extended theory formation
In 1963, the mathematician Roy Kerr described a solution for a rotating black hole with the Kerr metric. Until then, the terms black stars or frozen stars were used - the latter as a metaphor for the fact that, according to the theory, time seems to stand still at the edge of the black hole as seen from outside due to gravitational time dilation.
The term "black hole" is first attested in 1964 in a report by science journalist Ann Ewing on an American Association for the Advancement of Science symposium on the various end stages of stars. The author listed Hong-Yee Chiu as the organizer and Alastair Cameron, Charles Misner, Volker Weidemann, and John Beverly Oke as speakers, without naming the originator of the term. The term was established in 1967 after John Archibald Wheeler sought a replacement for the long expression "gravitationally completely collapsed object" at a conference and took up the suggestion of an unknown listener.
This was followed in 1971 by the discovery of Cygnus X-1, the first observable candidate for a black hole. In the theoretical field, Jacob Bekenstein established black hole thermodynamics in the early 1970s by assigning an entropy to the surface of the event horizon (Bekenstein-Hawking entropy). This was supported by Stephen Hawking's discovery (1974) that black holes emit radiation, Hawking radiation. At the same time, a connection was made from general relativity to quantum field theory.
In the 2000s, a trend developed to experimentally investigate phenomena not directly accessible to observation, such as Hawking radiation at black hole analogues, which are not gravitational systems but acoustic or electromagnetic or optical ones.
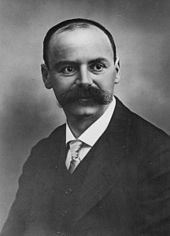

Karl Schwarzschild
Physical description
Metrics for black holes | ||
static | rotating | |
uncharged | Schwarzschild metric | Kerr metric |
loaded | Reissner-Nordström metric | Kerr-Newman metric |
|
Dynamics of emergence
In general, the mass of a body always results in gravitational forces. If the mass is limited to a small enough volume, the body will hold itself together: The gravitational force causes the body to compress. Normally, there are counterforces inside that stop further compression, leading to a balance between gravity and the counterforces. The counterforces can be thermodynamic pressure, repulsion between atoms or nucleons, or Fermi pressure, depending on the size of the object. The final stable mass limit is about 1.5 to 3.2 solar masses (Tolman-Oppenheimer-Volkoff limit); for objects that are lighter, the degeneracy pressure in matter that is in a degenerate state can successfully counteract gravitational collapse.
When a critical density is exceeded, the counterforces are no longer sufficient to compensate for gravity. A gravitational collapse is the result: the gravitational force increases faster than the counterforces resulting from the repulsion of the particles. As a result, the process accelerates itself. The mass collapses to a vanishing volume. The ever-increasing gravitation locally distorts space and the passage of time, in such a way that - viewed from a sufficient distance - the collapse slows down, the light rays emitted by the event become increasingly low in energy, and the volume never contracts to a single point.
Black holes can form from massive stars at the end of their stellar evolution. Stars of the main sequence above about 40 solar masses end up as black holes via the intermediate stages Wolf-Rayet star and supernova. Stars with masses between about 8 and about 25 solar masses, and all massive stars with high metallicity, end up as neutron stars. If their mass is between about 25 and about 40 solar masses, black holes can be formed by backfall of the material blown off during the incomplete supernova.
Gravitational effects
Since the mass is preserved, the density of the body grows beyond all limits. Such bodies bend the space-time around themselves so strongly that one could speak descriptively of a hole in the structure of the space, one calls them however more exactly singularity. The singularity is surrounded by a space-time region from which neither matter nor information can escape. The boundary of this region is the so-called event horizon, the distance of the event horizon from the singularity is the so-called Schwarzschild radius.
The event horizon is not a physical entity, it only denotes a place or more precisely a boundary surface. An observer falling through the event horizon would therefore not notice anything himself. However, relativistic effects (general relativity) cause a body viewed by a second, distant observer to take an infinitely long time to reach the event horizon due to time dilation, increasingly appearing in red-shifted light and becoming less luminous.
The gravitational field in the outer space of spherical, non-rotating and electrically uncharged bodies is described by the Schwarzschild metric. It applies not only to black holes, but to all bodies with these properties, and is usually a good approximation for stars or planets due to their low rotational speed. The size of the Schwarzschild radius for a black hole of one solar mass is about 2.9 kilometers, for an object of one Earth mass about 9 millimeters.
It is a common misconception that the gravitational field of a black hole, or the curvature of space and time it produces, is extraordinarily strong at common distances. Since both black holes and stars are described by the same metric, nothing would change in the gravitational field in the solar system if the Sun were replaced by a black hole of equal mass. Apart from the absence of sunlight, only in the immediate vicinity of the black hole (within approximately the previous core radius of the Sun) would there be an enormous increase in gravitational acceleration.
Rotation
The rotating black hole is a more general form of this astrophysical phenomenon. Rotating black holes are those that have an intrinsic angular momentum. Like all black holes, they cause a correspondingly large change in the geometric structure of space and time, due to their enormous gravitational pull (see spacetime curvature). In the case of a rotating black hole, however, the singularity takes on a circular or annular shape and sweeps space-time around it instead of merely curving it: Space is rotated along with it in the direction of the black hole's rotation. This kind of spacetime curvature does not appear in a black hole at rest, but in rotating black holes it occurs additionally outside the event horizon, so to speak, with the shape of a rotational ellipsoid flattened at the poles. All objects around a rotating black hole are also rotated, precisely because space-time itself also rotates.
To an observer standing still relative to his surroundings, it would seem as if the whole universe were rotating around him. This effect decreases strongly with distance. But up to a certain distance (the so-called static limit), in an area called the "ergosphere", the rotational speed is so high that all objects (and also energy such as light rays) would in turn have to be faster than light in order to compensate for the rotational speed, i.e. not to rotate along with it. The angular velocity of a particle at the actual event horizon corresponds exactly to the rotational velocity of the black hole. Towards the outside, the angular velocity of the particle decreases, but its orbital velocity always has a component in the direction of rotation of the black hole. This does not mean that its intrinsic velocity is greater than the speed of light, but that there can be no non-rotating particles within the ergosphere. This frame dragging is an extreme case of the Lense-Thirring effect, known since 1918. A special feature of the ergosphere is that the kinetic energy in this region can be negative from the point of view of an outside observer. A particle that is in the ergosphere can therefore decay into two particles in such a way that the kinetic energy of one of them is greater than that of the original particle. The particle in question can leave the ergosphere while its complement with negative kinetic energy (without further interaction) necessarily crosses the event horizon in finite proper time. The energy seemingly generated from nothing is extracted from the rotational energy of the black hole. This mechanism for energy generation was first proposed by Roger Penrose.
The extent of the ergosphere depends on the polar angle (corresponding to the complementary angle of latitude on Earth): At the poles of the rotating black hole, the static boundary coincides with the event horizon; in the equatorial region, it extends to a distance of at most twice the Schwarzschild radius, which depends on the black hole's angular momentum. The angular momentum of a black hole is thereby bounded, as will be described below.
Some observations, for example of extremely fast matter jets leaving the region outside the event horizon perpendicular to the accretion disk, are described by effects that can only occur within an ergosphere or in its presence. From general considerations of conservation of angular momentum, one can conclude that all black holes rotate, at least at the time of their formation. But of course, only very rapidly rotating black holes exhibit strong effects of the phenomena known as frame dragging. On the other hand, any rotating mass, regardless of the occurrence of an event horizon, so including planet Earth, twists the surrounding spacetime. These effects at the earth should be quantified by measurements for example with the help of the LAGEOS satellites. First results from 1997 were still so close to the range of measurement inaccuracy that they were controversially discussed, only a repetition of the measurement in 2004 with the satellite Gravity Probe B confirmed the facts.
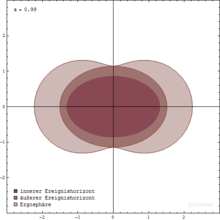

Ergosphere and event horizons of a rotating black hole (the inner event horizon is only a mathematical finding; the outer event horizon is the physically occurring event horizon)
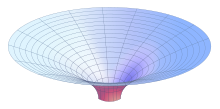

Outer Schwarzschild solution
Questions and Answers
Q: What is a black hole?
A: A black hole is a region of space from which nothing, not even light, can escape. It starts existing when spacetime gets curved by a huge mass and has an event horizon that anything inside cannot leave.
Q: Why are black holes black?
A: Black holes are black because they absorb all the light that hits them and reflect nothing, just like a perfect black body in thermodynamics.
Q: How do people find black holes?
A: People find black holes by tracking the movement of stars that orbit somewhere in space or when gas falls into a black hole and heats up and becomes very bright, which can be seen with telescopes on Earth or Earth-orbiting telescopes.
Q: Are there supermassive black holes?
A: Yes, astronomers have found evidence of supermassive black holes at the center of almost all galaxies. In 2008 astronomers found evidence that a supermassive black hole of more than four million solar masses is near the Sagittarius A* part of the Milky Way galaxy.
Q: Does quantum mechanics affect how we view black holes?
A: Yes, under quantum mechanics,black holes have a temperature and emit Hawking radiation, which makes them slowly get smaller.
Q: What happens inside a black hole?
A: Inside a black hole the rules of physics are very different than what we experience here on Earth.